advertisement
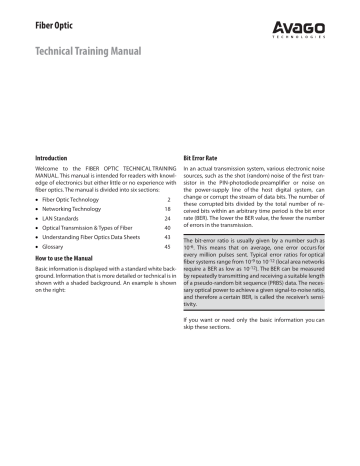
Fiber Optic
Technical Training Manual
Introduction
MANUAL. This manual is intended for readers with knowledge of electronics but either little or no experience with fiber optics. The manual is divided into six sections: x
Fiber Optic Technology x
Networking Technology x
LAN Standards x
Optical Transmission & Types of Fiber
2
18
24
40 x
Understanding Fiber Optics Data Sheets 43 x
Glossary 45
How to use the Manual
Basic information is displayed with a standard white background. Information that is more detailed or technical is in shown with a shaded background. An example is shown on the right:
Bit Error Rate
In an actual transmission system, various electronic noise sources, such as the shot (random) noise of the first transistor in the PIN-photodiode preamplifier or noise on the power-supply line of the host digital system, can change or corrupt the stream of data bits. The number of these corrupted bits divided by the total number of received bits within an arbitrary time period is the bit error rate (BER). The lower the BER value, the fewer the number of errors in the transmission.
The bit-error ratio is usually given by a number such as
10 -6 . This means that on average, one error occurs for every million pulses sent. Typical error ratios for optical fiber systems range from 10 -9 to 10 -12 (local area networks require a BER as low as 10 -12 ). The BER can be measured by repeatedly transmitting and receiving a suitable length of a pseudo-random bit sequence (PRBS) data. The necessary optical power to achieve a given signal-to-noise ratio, and therefore a certain BER, is called the receiver’s sensitivity.
If you want or need only the basic information you can skip these sections.
2
Fiber Optic Technology
A communication link can be made with a variety of media, including twisted-wire pair, coaxial cable, RF (radio frequency) or IR (infrared) wireless signal transmission, or fiber-optic cable.
Fiber-optic systems use pulses of light traveling through an optical fiber to transmit data. This method offers many advantages over copper wire, some of which are not available with other technology: x Complete input/output electrical isolation x No electromagnetic interference (EMI) susceptibility or radiation along the transmission media x Broad bandwidth over a long distance x Light-weight, small-diameter cables x Equal to the cost of copper wire and connectors
(except when copper wire is already installed)
Feature
Electromagnetic
Interference
(EMI)
High Bandwidth
Voltage Isolation
Voltage Insulation
Weight and Diameter
Benefit
Because optical fiber transmits light rather than electrons, it neither radiates EM (electromagnetic) fields nor is it susceptible to any
EM fields surrounding it. This is important in a number of applications:
• Industrial control, where cables run along factory floors in proximity to electrically noisy machinery. The optical medium permits the control signals to be carried error-free through the electrically noisy environment.
• Telecommunications equipment manufacturers use optical fiber because it eliminates cross talk between the telecommunication lines.
• Financial institutions and gaming equipment manufacturers require data security. Tapping into a fiber cable without being detected is extremely difficult.
Optical fiber has a relatively high bandwidth in comparison to other transmission media. This permits much longer transmission distances and much higher signal rates than most media. For example, all undersea long-haul telecommunications cable is fiber-optic. This technology is allowing worldwide communications (voice, video and data) to be available mainstream. With new technologies, such as VCSEL transmitters, parallel optics and wavelength division multiplexing (WDM), services such as video-ondemand and video conferencing will be available to most homes in the near future.
Fiber isolates two different voltage potentials. and insulates in high-voltage areas. For example, it will eliminate errors due to ground-loop potential differences and is ideal for data transmission in areas subject to frequent electrical storms, and presents no hazard to the field installer.
A 100 meter coaxial cable transmitting 500 megabits of data per unit time is about 800% heavier than a plastic fiber cable and about 700% heavier than a hard-clad silica cable, all of equal length and transmitting at the same data rate.
PGLDPHWHU
KDUGFODGVLOLFD+&6
The relative diameters of various types of fiber cable and coaxial copper cable for the same distance and data rate are shown in
Figure 1.
PGLDPHWHU
PXOWLPRGHJODVV
PGLDPHWHU
VLQJOHPRGHJODVV
PPGLDPHWHU
FRD[LDOFRSSHUZLUH
ZLWKPPGLDPHWHUMDFNHW
PPGLDPHWHU
SODVWLFRSWLFDOILEHU32)
Figure 1.
The Fiber-Optic Link
A basic link has three main components, each with a specific function. The transmitter contains a light-emitting diode (LED), laser diode (LD), or vertical cavity surfaceemitting laser (VCSEL) that converts an electrical current into an optical signal. The receiver contains a photodiode that converts the light back into an electrical signal and an amplifier that makes the signal easier to detect. The fiber-optic cable carries the optical signal between them.
/('
7UDQVPLWWHU
Figure 2.
&DEOH
3KRWRGLRGH
5HFHLYHU
Emitters
The two major groups of optical emitters for communication links are light-emitting diodes (LEDs) and lasers. The
VCSEL (Vertical Cavity Surface- Emitting Laser) is often categorized separately because of its similarities to both lasers and LEDs.
Emitters are made from semiconductor compounds. Elements in the third column of the periodic table combined with elements in the fifth column (known as “III-V” compounds--GaAs is one example) emit particles of light
(photons) when subjected to an electric current.
Using the right combination of elements, and the right processing parameters, engineers can produce a variety
The most common wavelengths for LED emitters are 650 nm for plastic fiber; 850 nm and 1300 nm for multi-mode glass fiber. Surface-emitting LEDs (SLEDs) are inexpensive to manufacture because they emit light from their surface and so require no precision cleaving. LEDs can also be made to emit light from the edge, producing a more narrow, collimated beam that can launch into single-mode fiber. Edge-emitting LEDs are called ELEDs.
Lasers
Lasers are devices that efficiently convert electrical power to optical power due to a process called stimulated emission. Photons produced by a semiconductor laser are initially trapped in a cavity and bounce off reflective surfaces at each end. They then repeatedly pass through an active gain region, where on each pass they stimulate the production of additional photons. Eventually the power in the cavity increases enough so that a very bright, coherent, collimated beam of light is emitted from one end of the cavity.
The gain medium determines the properties of the output light, including wavelength, output power, and whether the light exits in pulses or in a continuous wave.
Semiconductor lasers are devices with a unique structure that makes them more efficient.
1. Laser Diodes
In Laser diodes, the active gain material is a speeds (how fast the emitter can be turned on and off ) of the emitted light.
By optimizing its wavelength, the light can be efficiently coupled into the appropriate type of fiber when combined with an optimally designed optical sub-assembly
(OSA). The wavelengths span the visible and infrared spectrums up to 1550 nm. Typically, wavelengths 1300 nm and above are considered to be long wavelength
(LWL) and those below 1300 nm are considered to be short wavelength (SWL).
active region is very small and the input current is relatively low, laser diodes output optical power in milliwatts. By contrast, some medical lasers and industrial lasers output power in megawatts.
Because laser diodes emit light from the edge of the die, they are more difficult to manufacture and handle than LEDs. These devices must be cleaved, and mounted on their sides. Furthermore, it is more difficult to align the laser to the connector ferrule than it is to align an LED to the connector ferrule. For these reasons, lasers are more expensive than LEDs. Laser diodes are commonly found in 1300 nm and 1550 nm wavelengths for communications, and 980 nm and
1480 nm for pumping lasers.
LEDs
LEDs are diodes made of III-V material, which emit light.
As electrons pass through an active region of semiconductor material, they cause photons to exit. The light produced by an LED has a pure spectrum and is relatively brighter than a standard light-bulb emitter. The LED can be turned on and off at speeds which make data communications possible.
2. VCSELs
A type of laser that has recently emerged in the communications market is the vertical-cavity surface- emitting laser (VCSEL). This laser behaves as a laser diode, but can be manufactured similarly to an LED, because it emits light from the surface. This makes the VCSEL very attractive to the market due to its performance/ price ratio. VCSELs are being produced at 650 nm and
850nm, with 1300 nm to be produced in the near future.
3
LED Operation
Light-emitting diodes operate in the same way as diodes made from silicon or germanium: they allow glass optical fiber. Glass fiber loses a minimum of light at wave-lengths around 1350 nm, which is within the spectral band of an InGaAsP LED.
Laser Operation
current from flowing in the reverse direction. Current flowing through the diode in the forward direction generates a near-constant voltage across the diode, which is primarily determined by the material from which the diode is made. For example, diodes made from silicon have forward voltages of about 0.7 V and LEDs have forward voltages of around 1.3 V to 2.5 V. The forward current flowing through the LED generates light.
A laser (Light Amplification by the Stimulated Emission of
Radiation) operates in a manner similar to an LED. The difference is that the LED has spontaneous output only when forward biased. The laser begins with spontaneous output at low forward currents, increased to a point known as the Threshold Current level. Biasing above the threshold current causes the device to reach the stimulated emission region of operation.
The advantage of the laser is that it’s stimulated emis-
The light output of an LED is nearly proportional to the magnitude of its forward current. Therefore, controlling the forward current controls the light output of the
LED.
The circuit in Figure 3 shows one of the simplest ways to drive an LED. The LED current is determined by the difference between the input voltage and the forward voltage of the LED, divided by the series resistance. It is the current through the LED that controls the light output.
core optical fiber is typically far higher than that of an LED. Typically the laser also has a narrower output optical spectra, thus providing better performance at high bit rates over long distances. The disadvantage is that the threshold current is temperature dependent and circuit designers must take this into account. The added circuit complexity increases the cost of the laser transmitter.
5
,
)
Fabry-Perot Lasers
A FP laser has two parts: a semiconductor optical amplifier that provides gain and mirrors to form a resona-
9
LQ
9
) amplifier is formed by applying current to a lower-
Figure 3.
LED Materials
,
)
9
LQ
5
9
)
Numerous elements and compounds can produce however, are practical for LED devices. At the present time, most commercially available LEDs are made from combinations of gallium (Ga), arsenic (As), phosphorus
(P), Aluminum (Al), and Indium (In).
Certain materials can be made to produce a range of wavelengths by adjusting the relative proportions of the constituent elements. It should be noted that
LEDs actually emit light over a narrow range of wavelengths, not just at a single wavelength. For example, an
820 nm LED might actually emit light from about 800 to
840 nm, with the peak intensity at 820 nm.
form an optical waveguide to direct the light that is produced. Light radiates from the laser in a narrow-angled cone. As the current increases, the optical amplifier gain increases. When the amplifier gain equals the mirror loss, the lasing threshold is reached; the laser begins to oscillate above threshold current. FP lasers can produce many mW of output optical power and can be modulated to very high rates by varying the current to the laser diode. VCSELs
Vertical cavity surface-emitting lasers (VCSELs) emit light perpendicular to the top plane of a semiconductor wafer.
The VCSEL uses a Bragg reflector, a multi-layer dielectric mirror composed of alternate layers of high and low index of refraction material. For data communication applications, the diameter of the laser is made large enough to support multiple modes. This causes the central lasing peak to spectrally broaden. The wider spectral width is often designed into VCSELs to avoid mode-selective loss in multi-mode fiber applications.
The material used depends on the application. For example, plastic optical fiber has a minimum loss of transmitted light at wavelengths around 650 nm. There-fore, an
LED made from GaAsP would be best suited for use with plastic fiber. AlGaAs is also a good material for use with
The major advantage of the VCSEL design is ease of manufacture, simplified on-wafer testing, and simple packaging, resulting in lower costs. The device has higher output power and higher modulation rates than SLEDs (Surface
Light-Emitting Diodes).
4
Detectors
Detectors are typically PIN photodiodes that function exactly the opposite of an emitter diode: they absorb photons and convert them into electrons. Photodetectors can be made of either pure silicon, or a III-V compound, depending on the wavelength requirements. The detector must be able to detect the same wavelength emitted by the transmitter, or the link will not operate. The detector is always followed by a pre-amplifier (preamp) that converts the current coming from the diode to a voltage, which is used by most amplification schemes.
Other detectors include avalanche photo-diodes, which are more complex to operate due to their control circuitry, and silicon photo-detectors, such as photo-transistors or photo-FETs.
One important property of a receiver is its sensitivity. This property defines how small a signal the receiver is able to detect. The receiver must be able to detect a signal over the top of any electromagnetic noise that may be interfering with the system. A careful design of the preamp the receiver, can improve the sensitivity.
The importance of understanding this technology lies in how it relates to two important specifications of a fiberoptic link: distance and data rate. The first step is to determine the distance over which the data needs to be transferred and the data rate at which it is being sent.
Some applications will be defined by industry standards, which clearly state specific distances and data rates. Other applications will be proprietary, and you will have to work closely with the customer to determine the details.
The index of refraction of the core must be larger than that of the cladding for the light to travel through the waveguide:
(n core
> n cladding
provides total internal reflection)
For some glass fibers, the cladding is SiO
2
(glass) and the core has dopants (such as germanium) to increase the index of refraction.
The light travels through the waveguide in paths called modes. Each ray of light has a different mode, which creates a crowded waveguide. Not all of the modes that exit a light source are transmitted through the waveguide. A specific angle, the acceptance angle, serves as a cutoff for the light modes.
/RVW
Figure 5.
/RVW
&ODGGLQJ
&RUH
&ODGGLQJ
7UDQVPLWWHG
7UDQVPLWWHG
All light rays that enter the fiber at an angle greater than this critical angle, α, exit through the cladding and are lost. This angle can be calculated based on the value, n, of the core and cladding, and is usually represented as the numerical aperture, NA, a standard specification of any fiber. The NA is defined in Figure 6.
Fiber as an Optical Waveguide
Reflected light
The most basic optical waveguide is the step-index fiber, which consists of two layers, a core and a cladding. These layers are made of materials that have different optical transmission properties. One important property of these materials is the index of refraction (n), a material constant that determines the direction of the light through the material.
_
&ODGGLQJ
&RUH
&ODGGLQJ
1$ VLQ _ Q
FRUH
< Q
FODG
&ODGGLQJQ
Figure 6.
&RUHQ
Figure 4.
5
Bandwidth
Bandwidth determines how much information can be transmitted through a waveguide at one time. An analogy would be the speed of a fluid flowing through a pipe and, in fact, optical fibers are often called “light pipes.” One of the reasons that optical transmission is more desirable than electrical transmission is because optical offers a much wider bandwidth. This is due to the properties of light versus electricity.
The bandwidth of a transmission medium is limited by an effect called dispersion. This means that the input signal is spread out or distorted as it passes through the medium and has a wider or different shape at the receiver. The more dispersion in a signal, the shorter the time between the unique data bits. If they get close enough to each other, they can overlap, causing errors in the signal, as illustrated in Figure 7.
Figure 7.
Dispersion
In an optical medium, such as fiber, there are three types of dispersion: chromatic, modal, and material.
Chromatic dispersion results from the spectral width number of different wavelengths that are emitted from the LED or laser. The smaller the spectral width, the fewer the number of wavelengths that are emitted. Because therefore contribute to modal dispersion. One way to reduce modal dispersion is to use graded-index fiber.
Unlike the two distinct materials in a step-index fiber, the graded-index fiber’s cladding is doped so that the refractive index gradually decreases over many layers. The corresponding cross-sections of the fiber types are shown in Figure 9.
lengths (higher frequencies) these longer wavelengths will arrive at the end of the fiber ahead of the shorter ones, spreading out the signal.
One way to decrease chromatic dispersion is to narrow the spectral width of the transmitter. Lasers, for example, have a more narrow spectral width than LEDs.
&URVV
6HFWLRQ
0XOWLPRGH
*UDGHG,QGH[
&RUH
&ODGGLQJ
0XOWLPRGH
6WHS,QGH[ 6LQJOHPRGH
5HIUDFWLYH
,QGH[
3URILOH and therefore, does not contribute to chromatic dispersion.
Modal dispersion deals with the path (mode) of each light ray. As mentioned above, most transmitters emit many different modes. Some of these light rays will travel straight through the center of the fiber (axial mode) while others will repeatedly bounce off the cladding/ core boundary to zigzag their way along the waveguide, as illustrated in Figure 8.
/LJKW
3DWK
Note: Shading is used only to make the illustration clearer
Figure 9.
With a graded-index fiber, the light follows a more curved path. The high-order modes spend most of the time traveling in the lower-index cladding layers near the outside of the fiber. These lower-index core layers allow the light to travel faster than in the higher-index center layers.
Figure 8.
+LJKRUGHU0RGHORQJHUSDWK
&ODGGLQJ
&RUH $[LDO0RGHVKRUWHVWSDWK
/RZRUGHU0RGHVKRUWHUSDWK
&ODGGLQJ
The modes that enter at sharp angles are called higtravel through the fiber than the low-order modes and longer paths of these high-order modes. A good waveguide design appreciably reduces modal dispersion.
Modal dispersion can be completely eliminated by using a single-mode fiber. As its name implies, single mode fiber transmits only one mode of light so there is no spreading of the signal due to modal dispersion. A monochromatic laser with single-mode fiber completely eliminates dispersion in an optical waveguide but is usually used in very long distance applications because of its complexity and expense.
6
&ODGGLQJ
+LJKRUGHU0RGHVIDVWHU
The flat line is where the fiber does not contribute to any limiting effects. The sloping line is where the data rate decreases as the distance increases. Finally, there is a cut-off point, the vertical line, at which the receiver can no longer detect the signal.
Different types of fiber and different emitter wave-
&ODGGLQJ
*UDGHGLQGH[)LEHU
/RZRUGHU
0RGHV
VORZHU
Note: Shading is used only to make the illustration clearer
Figure 10.
this curve, so you must take all components of the link into account when determining a solution. Generally, distance versus data rate graphs and tables are available for most Avago general-purpose fiber-optic components.
The amount of information that an optical waveguide can transmit is usually specified by the band-
Table 1 compares the major specifications of fiber-optic cable.
Table 1.
bandwidth- distance product to about 20 MHz-km. In gradedindex fibers, pulse broadening is minimized at a specific operating wavelength.
The attenuation of the fiber that you choose may limit the distance over which the data can be sent. The dispersion effects of the link can limit both the distance and the data rate. It is generally a compromise between the two: the longer the distance, the lower the data rate that can be sent, and vice versa. A typical distance versus data rate plot appears as shown in Figure 11.
Specification
Bandwidth-Length-
Product (MHz-km)
Plastic/Plastic Glass/Plastic Glass/Glass
3
Core Diameter (μm) 350 - 000
Transmission Dist.
Short
Attenuation (dB/km) 250
Core/Cladding
20
125 - 600
Medium
6
300-1500
50 - 100
Long
4
Numerical Aperture 0.50
Wavelength (nm) 650
0.37
800
0.30
1300 - 1550
'DWD5DWH
0%G
'LVWDQFH
P
Figure 11.
Attenuation
As light pulses travel through the fiber, they lose some of their photons, which decreases their amplitude. This is know as attenuation, the other major factor that limits signal transmission (light output power) and consequently, reduces the length of the fiber. Attenuation is usually specified in decibels per kilometer or per meter (dB/km or dB/m). Attenuation of optical power in a fiber has three main causes: scattering, absorption, and bending. Attenuation varies over the light spectrum.
7
Rayleigh Scattering
A ray of light is partially scattered into many directions by microscopic variations in the core-cladding interface. The angle of some of the light rays impinging at these variations is changed enough so that they are refracted onto different paths and do not experience TIR (see Snell’s
Law). For this reason, some light energy is lost. Scattering is responsible for up to 90% of the total attenuation. Rayleigh scattering causes about a 2.5 dB/km loss at 820 nm but less than 1 dB/km in wavelengths over 1000 nm.
Absorption
Impurities in the glass, which absorb light energy and turn photons into phonons (heat), include ions of copper, iron, cobalt, chromium and the hydroxyl (OH ) ions of water. The OH-ions of water and the molecular resonance of SiO
2
are the principal reasons that light energy is absorbed by the fiber.
Table 2.
Medium
Copper Wire
Plastic Fiber
Glass Fiber
Delay (ns/m)
4.0
4.8
5.0
The extra delay in travel time through a fiber (compared to copper) does not necessarily reduce the bandwidth; however, failure to allow for it in system design can have an adverse effect on message protocol.
When there are parallel logic paths using individual parallel links, the signals can arrive at the other end of the links at different times because the transit time for each link is different. If this happens, individual link delays might affect the performance of the circuit when all parallel paths are strobed at once. The strobe edge should be timed to just follow the longest individual transition delay and to precede the shortest individual transition delay.
Bending
Two types of bending exist: micro-bending and macroin the fiber geometry, such as, rotational asymmetry, variations of the core diameter, and course interfaces between the core and cladding caused by pressure, tension and twist. Macro-bending is due to fibers curved around diameters of about a centimeter, causing less than total reflection at the core-to-cladding boundary.
Bending loss is usually unnoticeable if the diameter of the bend is larger than 10 cm.
In multi-mode fibers, a large number of modes is always present; and each mode in a fiber is attenuated
Pulse-Width Distortion (PWD)
Pulse-width distortion refers to the time difference between the delay of a low-to-high edge and the delay of a high-to-low edge of the output signal waveform.
Ideally, low-to-high and high-to-low transitions should ence between them is distortion, and this can limit the signal rate.
Wavelength Division Multiplexing (WDM)
The data rate for a single optical channel eventually reaches a limit due to chromatic and other types of mode fibers this effect is not as much of a problem.
be increased by adding multiple wavelength channels.
Other Factors
Optical power loss in a fiber can also be caused by: x light reflected off the ends of the fiber by differences in the refractive indices of the core and air at the interfaces (Fresnel reflection) x poor finishing of the fiber ends, which scatters a portion of the light x variations in the diameters and cross sections of the core and cladding along the length of the fiber length channels onto a single fiber with low loss, then later separates them at the receiver end of the link.
'DWXP
'DWXP
'DWXP
'DWXP hhhh hhhh hhhh hhhh
0XOWLSOH[HU
$PSOLILHU
:DYHOHQJWK
&KDQQHO
$GGHU'URSSHU
$PSOLILHU hhhh hhhh hhhh
'HPXOWLSOH[HU hhhh
'DWXP
'DWXP
'DWXP
'DWXP
Propagation Delay
Propagation delay is the length of time it takes the output of a device to go from one state to another state, either low to high or high to low, after having been stimulated to change at the input. It is also a measure of the time required for a signal or pulse to travel through a medium. Table 2 lists the typical propagation delay times in ns/m for various media.
Figure 12. Wavelength division multiplexed fiber-optic link
In the past, when signals needed to be routed to a specific location, the optical signal was detected and routed electronically. WDM systems allow the optics to route the optical signal. These systems can also add and drop certain wavelength channels from a fiber while simultaneously sending on other channels.
8
Each type of fiber has a different attenuation versus wavelength curve, none of which is linear or exponential, but rather a complicated series of peaks and valleys.
The attenuation of the light through a glass optical waveguide has been decreased over the last 25 years to less than 0.02 db/km and scientists are trying to decrease the attenuation even more.
Figure 13 depicts the attenuation for a specific type of plastic optical fiber. As the graph shows, certain wavelengths are ideal for transmission because they fall in the valleys of the curve. For this type of plastic fiber, the best suited wavelengths are 570 nm and 650 nm. For most glass fibers there are valleys around 850 nm, 1300 nm, and 1550 nm. For other types of fiber, such as HPCS (Hard
Plastic Clad Silica) fiber, the attenuation curve is relatively flat over a wide spectral range.
Indirect Measurements
Cutback Method
The attenuation of a fiber can be measured by transmitting a signal through it and measuring the power at the opposite end. The fiber is then cut near the input end without changing the launch conditions and the power is measured again. The loss per unit length can be calculated from the difference between these two values.
Substitution Method
A short length of reference fiber is compared with the attenuation of the fiber being tested. Both the cutback and substitution methods have drawbacks. The attenuation measured by the cutback method varies according to the numerical aperture and the spectral bandwidth of the source. In the substitution method, the coupling losses of the fiber being tested and the reference fiber may be different, so the value derived may not be correct.
:DYHOHQJWKQP
Figure 13.
This feature makes these types of fibers useful for a variety of applications.
Attenuation loss in a fiber can be calculated by taking the logarithmic ratio of the output power (Po) to the input power (P i
): dB = 1 0 l og
1 0
Po
Pi
Direct Measurement
A more reliable technique for measuring attenuation in a fiber is optical time-domain reflectometry (OTDR). In this method, a brief signal (a short burst of optical power) is introduced into the fiber. When this signal encounters imperfections or discontinuities, some of the light is reflected by a beamsplitter (a glass plate at a 45 degree angle to the incident light or two prisms cemented together with metal-dielectric film between their faces) onto a detector, where it is amplified and displayed on an oscilloscope.
The ODTR display is a smooth curve for a continuous length of fiber and an irregular curve if discontinuities or splices exist. The advantage of OTDR is that it yields more accurate results.
9
Bit Error Ratio
In an actual transmission system, various electronic noise sources, such as the shot (random) noise of the first transistor in the PIN-photodiode preamplifier or noise on the
5-volt power-supply line of the host digital system, can change or corrupt the stream of data bits. The number of these corrupted bits divided by the total number of received bits within an arbitrary time period is the bit error ratio (BER). The lower the BER value, the fewer the number of errors in the transmission.
The bit-error ratio is usually given by a number such as 10
This means that on average, one error occurs for every million pulses sent. Typical error ratios for optical fiber systems range from 10 -9 to 10 -12 (local-area networks require a BER as low as 10 receiver’s sensitivity.
-12
-6 the fiber- optic transmitter and an entire system com-
.
). The BER can be measured length of a pseudo-random bit sequence (PRBS) data.
The necessary optical power to achieve a given signalto-noise ratio, and therefore a certain BER, is called the
(((((((
%,7(55255$7,2%(5
Figure 14. Receiver Threshold-to-Noise Ratio vs. BER
At any fixed temperature the total value of the receiver’s random noise plus the host system’s noise can be assumed to be a constant. So, the most obvious way to reduce the probability of error is to increase the amplitude of the optical signal applied to the receiver.
Another technique for lowering the error rate is to improve the receiver’s ability to reject electrical noise from the system it is in. One method is to use small
PIN diode pre-amps in the receiver’s first stage that do not function as efficient antennas. A second method, for extremely noisy applications, is to use PIN diode preamps in electrically conductive plastic or all-metal packages.
The worst source of noise is usually the host system’s power (usually +5 V) supply. The host system’s +5 prised of relatively noisy digital circuits. Simple and inexpensive power supply filters have been proven to work in a wide range of system applications. Simple, third-order pi power-supply filters are normally sufficient to protect the fiber-optic receiver from very noisy host systems, but in extremely noisy applications, additional power supply filtering might be needed.
Receiver sensitivity is specified at a BER; however, in practice, the BER is too low to be directly measured in a useful time period. For this reason, the transceiver is usually tested during an artificially shorter time at a much higher
BER. The resulting data is then fitted to a curve and the optical input power at the required BER is extrapolated.
Note:
The receiver’s signal-to-noise ratio determines the probability of error; small increases in this ratio will sharply reduce the probability of error. Optical budgeting is a design technique that takes system- and component-level parameters into account.
System-level parameters include: x transmitter output power (P
T
dBm avg.) x receiver sensitivity--the minimum optical power that the receiver requires to operate at the specified data rate and bit error ratio (P
R
dBm avg.) x fiber losses (caused by attenuation, bandwidth and insertion losses from connectors and are dependent on the type of fiber) x dynamic
Component-level parameters include: x
data x
bit-error ratio (BER) x
data
These two sets of parameters allow a designer to determine the distance and power budgeting for the fiber link.
Optical Power Budget
Power budget is the difference between the minimum sensitivity of the receiver. If optical power losses are greater than the worst-case power budget, the BER increases and the reliability of the link decreases.
10
Optical power expressed in μW or dBm, and optical attenuation is expressed in dB/m. The total loss budget is derived from the individual losses contributed by attenuation and other possible losses in the link.
The Optical Power Margin is
φ
M
= (φ
T
- φ
R
) - ( D
O
O + D
CC
).
Optical Power Margin = Optical Power Ratio - System
Losses.
Note:
For the fiber-optic cable to transmit and receive, the flux margin must be positive.
Each of these losses is expressed in decibels (dB) as
Pout
Loss = 1 0 l og
Pin where P in
and P out
are the optical powers into and out of the loss element.
Note: dBm is a unit used to describe optical power with respect to one milliwatt.
For example, if P
IN
= xμW), then
3 /RJG%P
+ :
The link loss budget considers the total optical power loss allowed between the light source and the photodetector, and allocates this loss to cable attenuation, connector loss, splice loss, and system margin.
The optical power budget is P
T
- P
R
, where P
T
is the power launched by the transmitter and P
R
is the power required by the receiver for proper operation. These values are usually expressed in dBm, so the difference is expressed in dB. If the losses between transmitter and receiver exceed the power budget, then the power at the receiver will not be adequate to assure proper operation.
In the following example, P
T
= -12 dBm and P
R
= -24 dBm.
Substituting these values into the equation:
P
T
- P
R
= -12 - (-24) = -12 + 24 = +12 dB, and so, the receiver has sufficient power to operate.
Eye Diagrams
An oscilloscope display of a long stream of random bits
(light pulses) is used to determine transmitter and receiver performance. Due to distortion of the waveform (by a variety of factors), the displayed light pulses do not align, but instead overlay one another and appear blurred, as shown in Figure 15.
The important point is that the center of the wider the opening, the less the distortion and jitter.
Figure 15.
Much information about system performance can be gathered from the eye pattern: x
The width of the eye defines the time interval during which the received signal can be sampled without error from inter-symbol interference x
Amplitude distortion in the data signal reduces the height of the eye opening. The smaller this dimension, the more difficult it is to detect the signal without error x
The height of the eye opening at the specified sampling time shows the noise margin or immu-
0D[LPXP
VLJQDOYROWDJH
9
6ORSHLQFUHDVHV
VHQVLWLYLW\WR
WLPLQJHUURUV
7KUHVKROG
Figure 16.
following equation:
V
1
Noise margin (per c ent) = X 1 00 per c ent
V
2
where V1 is equal to peak signal voltage and V2 is equal to the ideal signal voltage
11
%HVWVDPSOLQJWLPH
7LPHLQWHUYDOGXULQJ
ZKLFKVLJQDOFDQ
EHVDPSOHG
'LVWRUWLRQDW
VDPSOLQJWLPHV
1RLVHPDUJLQ9
'LVWRUWLRQDW
]HURFURVVLQJ
6 7
x Timing jitter is caused by noise in the receiver and pulse dispersion in the fiber. If the signal is meacates the amount of jitter x The lower the slope of the signal’s transition time, the more that the eye closes and the greater the increase in timing errors. As the slope approaches the horizontal, the possibility of error increases x If a truly random data stream passes through a linear system, all eye openings will be identical and symmetrical. Any non-linearities will produce asymmetry in the eye pattern
Parameters such as rise- and fall-times, extinction ratio, overshoot, undershoot, and pulse-width distortion can be observed.
fying pulse parameters individually. It is compact, conveying important information without placing undue restrictions on the data pulse. Limiting rise and fall times, pulsewidth distortion, overshoot, damping, undershoot, and so forth, is unwieldy and insufficient by comparison.
0DVNUHJLRQ
0DVNUHJLRQ
When an oscilloscope is triggered by the clock, the result is the classical “eye” pattern, as shown on the preceding page. Data are recoverable if the “eye” is
“open” above and below the threshold for a period of time greater than the “set-up” and “hold” time needed for subsequent decision devices or circuits.
Most serial communications standards now define Figure 17.
0DVNUHJLRQ
5HODWLYH7LPH8QLW,QWHUYDO
$EVROXWH7LPHV plate) for the serial data stream. The area from which the waveform is excluded is the mask.
The mask limits that specify the eye-pattern opening are an improvement over the older technique of speci-
As shown by in Figure 17, a mask consists of two parts:
1. defined regions on the oscilloscope screen where the waveform is not permitted to appear
2. definitions of the time and amplitude scales
TTL and PECL
ECL (Emitter Coupled Logic) is a non-saturating digital logic, characterized by high speed, low noise, and the ability to drive low-impedance circuits. ECL uses a negative power supply. PECL (Positive Emitter-Coupled Logic) is the standard ECL device powered by a positive power supply.
A typical application for ECL (Emitter Coupled Logic) is a subsystem that requires very fast data transmission, such as fiber-optic links. In this application, parallel data is serialized into a highbandwidth PECL data stream. At the other end of the link, the signal is converted back to relatively low-speed parallel data for further processing. This system of translating parallel to serial then serial to parallel minimizes the number of transmission lines required for interconnecting the subsystems.
The gigabit rate performance of current fiber-optic link designs demands the use of PECL circuits.
In Figure 18, the high-speed serial data from the SerDes
(serializer-deserializer) IC is PECL-compatible with the
Optical Transceiver. The low-speed Signal Detect output from the Optical Transceiver is PECL. The Host Protocol
IC, which uses signal detection, operates with TTL logic levels. It is converted from PECL to TTL using the Converter IC. The parallel data interface between the Host Protocol IC and the SerDes IC is TTL.
77/
+RVW
3URWRFRO
,&
77/
&RQYHUWRU
6HU'HV
3(&/
6LJQDO
'HWHFW
3(&/
2SWLFDO
7UDQVFHLYHU
)LEHU2SWLF&DEOH
Figure 18. Simplified Gigabit Ethernet Network Interface Card
12
Data Communication Links
A data communication link transfers information from one piece of electronic equipment to another. These links interconnect computers, peripherals, servers, and other individual devices. Busses inside these devices distribute the parallel data between the internal components
(memory chips, the micro-processor, logic devices, and so forth). To send this parallel data across a datacommunication link, it must first be transformed into a serial bit stream.
To do this, the transmitter, receiver and the supporting ICs must do more than merely convert current into light. Sometimes the manufacturer, for example, Avago, builds additional circuitry into the fiber-optic transmitter and receiver and sometimes it is instead the responsibility of other equipment designer and the integrated circuit vendors. The level of integration is different, depending on factors that will be discussed later.
'ULYH
&LUFXLWU\
5HFHLYH
&LUFXLWU\
Figure 19.
/('RU
/DVHU
2SWLFDO)LEHU
3KRWRGLRGH
A number of steps are needed to prepare this serial bit stream from the parallel word data and transmit it.
'DWD
%XV
%XV,QWHUIDFH
&LUFXLWU\DQG
6RIWZDUH
%XIIHULQJ
)UDPLQJ
(QFRGLQJ
&LUFXLWU\
0XOWLSOH[RU
6HULDO
6LJQDO
3URFHVVLQJ
'ULYH
&LUFXLWU\
7[
)LEHU2SWLF&DEOH
5[
5HFHLYH
&LUFXLWU\
&ORFN
DQG
'DWD
5HFRYHU\
5HDVVHPEO\
%XIIHULQJ
'HPXOWLSOH[RU
'HFRGLQJ
&LUFXLWU\
%XV,QWHUIDFH
&LUFXLWU\DQG
6RIWZDUH
'DWD
%XV
1HWZRUN,QWHUIDFH&DUG
Figure 20. Block Diagram of a Generic Fiber-Optic Data Communication Link
1HWZRUN,QWHUIDFH&DUG
Table 3.
Block Description
Bus Interface Interfaces directly with the data bus, recognizes requests to send data, pulls the data off the bus, and maps the data from the bus format over to the data communication link format. When the data link is receiving data, the bus interface notifies the local bus that information has been received and is going to be put on the bus. It then maps the data from the data link format to the specific bus format.
Buffering / Framing
Encoding / De-coding
Stores the parallel data from the bus in local memory. Partitions this large block of data into frames (small blocks); overhead
(additional frames) may be inserted. These overhead frames include address error tolerance, and LAN management information. All frames are then sent sequentially to the transmitter-encoding circuitry. When receiving data, this procedure is reversed and the original data is restored and placed in local memory. It remains here until the bus interface circuitry places it on the resident data bus.
Converts the data from an original value to a prescribed value, which when serialized, ensures that the link will function properly. Since this conversion is “prescribed,” the receiver side of a data communication link can convert the received signal back into the data’s original form.
Multiplexor/ Demultiplexor Serializes the parallel data (a data communication link can transmit only one data bit at a time along the same cable). The serial bit stream goes to the Serial Signal Processing circuitry. On the receiver side of the link--once the serial bit stream has been recovered--this data is converted back into parallel data words by the demultiplexor (DeMux).
Serial Signal Processing
Drive Circuitry
Changes the digital serial bit stream, if necessary, into a form appropriate for the transmission medium.
Drives the transmitter to send the serial signal, which is usually amplified. The drive circuitry may convert an input voltage signal to a current drive signal, which is sent to an LED or laser diode (both of which are current driven).
Transmitter Converts the electrical signal into a form appropriate for propagation through the transmission medium. For fiber optics, the signal is converted into light, which is launched into the fiber-optic cable.
13
Table 3. Cont.
Block Description
Transmission Medium
Receiver
Receive Circuitry
Clock and Data Recovery
Carries the signal from the transmitter to the receiver. The medium can be twisted-wire pair (TWP), coaxial cable (coax), fiber-optic cable (glass or plastic) or wireless (does not require a medium).
Detects the incoming signal and converts it to an electrical signal, which can be processed by the receive cir-cuitry.
Processes the signal from the receiver into a more useable form. Frequently the signal is converted from current to voltage
(by a trans-impedance amplifier) or amplified (by fixed and variable gain amplifiers) or wave-shaped (by an equalizer for copper cable transmission or quantizer for some fiber-optics transmission) or can undergo any combination of these procedures.
Reconverts the received and processed serial signal into the original serial bit stream. For this to happen, the clock and data recovery must first lock onto the frequency and phase of the incoming signal and extract the clock (by a phase-locked loop, or some other method). This clock is then used to check the incoming signal at every clock period. In this way, the original digital serial bit stream is recovered along with the clock (data rate at which the serial bit stream was sent).
Encoding
Encoding allows the data transmission link to be more efficient. Encoding merges the data and clock signals in a manner that allows a timing recovery circuit to reconstruct the clock and data signals at the receiver end of the data link. Without encoding, a clock signal would have to be sent via a second fiber link. Increased cost and possible timing skew problems could arise if an additional clock link were to be established. A number of encoding schemes are shown in the following table.
The basic unit of information in a digital system is the bit: a logical “one” or “zero.” A logical one is represented by a high voltage; a logical zero by a low voltage. In binary signaling, a “symbol” is either a high of low signal level that is held for a period of time. When a string of identical bits is transmitted, the voltage remains at the same level. Unencoded data has DC voltage offsets because this data can remain in the “logic 0” or “logic 1” state for an indefinite period. Digital systems use a clock to define the bit period in a constant-voltage transmission.
Data rates are often expressed in bit per second. The terms, megabits per second (Mb/s), megahertz (MHz), and megabaud (Mbd) are related as follows: the translation from bits per second to baud depends on the encoding scheme. If there is no encoding, bits per second equals baud. Baud is expressed in symbols/second. In binary transmission systems, the maximum fundamental frequency (Hertz) of the data is half the baud rate.
Type
Manchester
Description
Replaces each bit with 2 symbols
Mb/s
100
Mbd
200
MHz
100.0
Efficiency
Less
4B5B* Replaces 4-bit group with 5 symbols 100 125 62.5 More
5B6B Replaces 5-bit group with 6 symbols 100 120 60.0 More
*Note:
Another type is 8B10B, which replaces 8 bits with 10 symbols. The relationship between Mb/s, Mbd, and MHz remains the same for this encoding as well.
At the transmitter end of the link, serial binary data is coded to a signal suitable for transmission. At the receiver end of the link the digital signal is demodulated by a phase-lock loop (PLL), a circuit that synchronizes a local oscillator with the incoming signal. A PLL has three basic elements: a phase detector (PD), a loop filter (LF), and a voltage-controlled oscillator (VCO), as shown in the following circuit diagram, Figure 21.
,QSXW
6LJQDO
3KDVH
'HWHFWRU
/RRS
)LOWHU
9&2
Figure 21.
14
The phase detector compares the phase of a periodic input signal to the phase of the VCO. The voltage output of the PD is the difference in phase between the two inputs. This voltage is filtered by the LF and applied to the VCO. Control voltage on the VCO changes the frequency to reduce the phase difference between the input signal and the VCO. When the loop is locked, the
VCO is exactly equal to the average frequency of the input signal. Each cycle of input has only one cycle of oscillator output.
The number of symbols per second is expressed in baud. Although bit rate and baud may coincide, they are not the same. The fastest baud is defined as the reciprocal of the narrowest pulse width. Data rate is influenced by format, which is the method of encoding bits for transmission. Baud is a true measure of a system’s signaling speed because it is not dependent on the data format. Table 4 describes the various modulation codes used in data transmission.
Table 4. Standard Modulation Codes
Code Description
NRZ A logic-level code for serialized data. A “zero” is a low logic level and a “one” is a high logic level that does not return to zero between successive “ones.”
RZ
NRZI
A code in which “ones” are represented by a high logic level that returns to zero between successive “ones.” For this reason, the code requires a channel that can signal two symbols (high and low) per bit. Its main advantage is that it conserves power because energy is needed only for transmitting “ones.”
A code in which a “zero” is represented by an edge and a “one” is represented by the absence of an edge. Because the edges may be either rising or falling, inversion of the signal does not change the meaning of the code and it is therefore invertible.
Biphase-Mark A code requiring a channel capability of two symbols per bit. Each bit cell begins with an edge: then, for a “one” an additional edge occurs during the bit cell; for a “zero” there is no edge during the bit cell. Because there is always an edge at the beginning of the bit cell, clock recovery from this code is possible by either phase-lock or one-shot techniques. It is also invertible and has a duty factor exactly 50%.
Biphase-Space An invertible, self-clocking code, differing from biphase-mark only in that ’zeroes” have the additional edge during the bit cell. Both biphase codes are sometimes called “f/2f” because the additional mid-cell edge may be regarded as a cycle of a frequency that is twice that for the absence of the mid-cell edge. Duty factor = 50%.
Manchester A code in which a “one” has a high logic level at the beginning of the bit cell with high-to-low transitions at mid-cell; a “zero” starts at a low level with low-to-high transition at mid-cell. This code also requires a channel capability of two symbols per bit. Because there is a mid-cell transition in each bit, clock recovery is possible by either phase-lock or one-shot techniques. It is not invertible; the duty factor is 50%.
Miller An edge-type code in which each “one” in the serial data is encoded as a mid-cell edge. The “zeroes” either have no edge or are encoded as edges at the beginning of the bit cell. Because no edges occur at intervals less than one bit time, the channel capability required is only one symbol per bit. With edges occurring regularly at integral multiples of half of a bit time, clock recovery is possible with a phase-locked oscillator running at a frequency that is twice the data rate. The duty factor is generally 50%.
MFM* A code similar to Miller: impulses occur at intervals of 1.0, 1.5, or 2.0 bit times. For this reason, clock recovery is possible.
Because impulse duration is much less than bit time, the duty factor approaches zero but cannot exceed 50%.
*Modified Frequency Modulation
Electromagnetic Compatibility—EMC
EMC refers to the capability of electronic equipment or systems to be operated in the intended operational electromagnetic environment at their designed levels of efficiency. Specifically, EMC describes how the product known as electromagnetic interference (EMI), and also how the product’s performance is affected by immunity (sus-ceptibility to radiated energy), electrostatic discharge (ESD), and conducted power supply noise.
15
1. EMI
The first EMC area is radiated emissions, sometimes called electromagnetic interference or EMI. A radio or TV broadcast is an example of intentionally radiated electromagnetic energy. A computing device also radiates electromagnetic emissions, although it is not intended to (the radiation is an inherent by-product of the switching currents flowing in its conductors). Electromagnetic interference (EMI) describes the effect of unwanted radiation
Vcc-toground- current loops. These antennas are then driven by various energy sources within the system circuitry. Keep these antennas as small as possible. If they are long enough to be treated as transmission lines, then it is important that the line is terminated in its characteristic impedance. Antennas can also be formed by long traces that are driven by ground voltage noise and therefore radiate. Even a ground plane, when driven by a ground noise source can radiate. Often, cables can radiate energy when high-frequency currents flow through them.
Because cables are often the largest antenna around, they are usually the dominant source of radiation. Chassis and cable shields can also radiate if ground noise current or voltage drives them.
occurs when a changing current flows in a conductor.
The area near the conductor (antenna) is where either the electric or the magnetic field dominates the total radiated field.
Government agencies around the world regulate the amount of radiated electromagnetic energy emitted by various sources. Their intent is to allow any purposely transmitted radiated energy to be received without being interfered with by some other radiation source at around the same frequency.
Fiber-optic cables do not, however, radiate energy as wire cables do. Therefore, fiber-optic cables can help reduce radiated emissions if using wire cables is a problem. At FDDI and ATM data rates, the encoding/ decoding schemes, and the consequent additional circuitry needed to reduce the bandwidth and the emissions on a twisted pair wire cable, are not needed if a fiber-optic cable is used.
Most manufacturers want their systems to meet all of tions used around the world so that they can be sold in the US, in Europe or in Japan, without restrictions. Government agencies usually set their radiated emissions regulations to distinguish between two types of applications. One is factory or office (Class A) where a higher level of radiation can be tolerated; the other is the home
(Class B) where there are more TVs and radios and therefore less electromagnetic radiation can be tolerated.
If an antenna is completely enclosed in a sufficiently thick metal box, then no radiation will escape. If the box has an aperture, then some radiation will escape through it; the bigger the opening and the higher the frequency, the more radiation that escapes.
Any product that does not meet the pertinent emissions requirement for a particular country cannot be sells equipment that is found to violate the regulations can, if caught, face large fines and penalties. Also the manufacturer may be forced to withdraw the product facturer may be forced to withdraw the product from the market until the regulating government agency, for example, the
FCC (Federal Communications Commission) in the United
States is convinced that the radiated emissions of the modified product meet the required specification limits.
More accurately stated, the leakage through a single aperture in a metallic chassis is related to the longest dimension of the opening (d) and the wavelength (λ) of the radiating frequency. When wavelength is less than or equal to twice the longest aperture dimension, the attenuation of the aperture is 0 dB. The cutoff frequency of a solitary aperture occurs when λ = 2d. At frequencies less than cutoff, the attenuation of the aperture increases at a linear rate of 20 dB per decade. If the metal chassis box has multiple openings with identical longest dimensions d, then the attenuation of the shielding decreases by 10 log (N), where N equals the number of apertures. The preceding relationship holds true until N becomes large enough to reduce shield attenuation to 0 dB.
2. Electromagnetic Susceptibility
System design can affect radiated emissions in three ways.
The first way is in the choice of circuit components. Some generate more high-frequency energy than others do.
The second way relates to the effect of the antennas (that is, the circuit interconnections) that the high-frequency energy sources are connected to. The third way is by the shielding that the chassis box provides and by the cable shields that effectively reduce the amount of radiation that the antennas leak to the outside world.
Electromagnetic susceptibility (or immunity) of a product is defined as the effect of external electromagnetic fields on the performance of that product. The performance is measured in the presence of an external electromagnetic field relative to the performance with the electromagnetic field absent. Immunity and susceptibility refer to the same characteristics (immunity is the inverse of susceptibility).
An actual communication system contains various What is meant by immune, however, is left to be negotiated between vendor and customer. Based on some the other metal bodies in the circuitry that create the
16
of Avago’s customer inputs, Avago has standardized on a 10 V/m field strength to test fiber-optic transceivers.
Usually an antenna in the circuit will pick up the external field and then couple it into a critical circuit node where it appears as a noise signal. The susceptibility will depend on the frequency of the field because the receiving antenna gain varies with frequency and because the circuit noise rejection varies with frequency. The noise can be picked up directly at the sensitive node itself or can be conducted from another node (such as Vcc) that has a larger gain antenna connected to it.
nents, then the system can be manufactured without ESD damage.
In a finished system, ESD can still cause permanent product damage. Often, however, a more important problem is ESD disturbances to the system performance. An ESD performance disturbance could include lines on a CRT display, logic stuck in a locked state, or a larger number of bit errors than usual. These ESD disturbances can be accounted for in the system design to ensure that the product’s end user does not notice any drastic performance differences when an ESD occurs.
The usual effect of an external field on a fiber-optic receiver is to degrade the received bit error rate. The fiproduct. An external field can induce a signal on the antennas formed by the interconnections in the fiber-optic same phenomenon that causes an antenna to radiate an electromagnetic field when a voltage/current signal is applied to its inputs, will generate the same voltage/ current signal at those inputs, (now really the outputs), if the same antenna is placed within an identical, but externally generated, electromagnetic field. The antenna, formed by the Rx circuit inter-connections, picks up the external field and generates a signal. If the generated noise signal is conducted to a sensitive circuit node, the
If the components are enclosed inside a conductive or static-dissipating chassis box in the end product,
ESD is more likely to go to the conductive box than to some other non-conductive component. For example, if a plastic-nose, fiber-optic module is protruding from the chassis box, then ESD is more likely to be conducted to the section of the chassis box that is near the module nose than it is to be conducted to the insulating transceiver’s plastic-nose itself. If the chassis grounding is such that the ESD currents to ground flow on the chassis and do not flow inside the PCB component grounds, then ESD damage or ESD problems due to radiated fields caused by the ESD pulse will be reduced.
4. Conducted Noise
The fourth EMC area is conducted noise. Ideally a conducwhich increases the bit error rate. (In a perfect fiber-optic tor will carry only the desired signal. Practically, however,
Rx, the signal-to-noise ratio and the bit error rate are dithere is always some component of the actual signal on rectly related). So the external electromagnet-ic field can the conductor that is undesirable. This component is inject noise into the fiber-optic Rx and thus degrade the defined as noise. Conducted noise emanates from one bit-error rate (BER).
section of the product’s circuitry and is conducted to the section of the circuitry being observed.
3. Electrostatic Discharge
Certain non-conductive materials can either
There are three main components of conducted noise: donate charge (electrons) or acquire charge when in
1. the conducted noise generator
2. the path that the noise takes to conduct from the generator circuit to the receiving circuit charge can then transfer it to a conductive material either by direct contact or by inducing the opposite charge in the conductor. If this charged conductor contacts an earth ground (or any conductive body with a very large amount of stored charged available), a current will flow until that conductor’s net charge becomes zero. For example, if your skin is charged from walking across the carpet on a cold dry day and you then touch a grounded or large conductor, you may see a spark as your skin discharges, and feel a tingle in your finger, as the current flows. The characteristics of the ESD current depend on the amount of charge stored and on the impedance of the circuit that discharges it (to ground).
ESD can affect a product during its manufacturing or during its operating lifetime. If all the ESD events are controlled during manufacturing so that they lie safely within the limits of the most ESD-sensitive compo-
3. the sensitivity of the receiving circuit to this noise
So, conducted noise problems could be eliminated by eliminating the noise source, removing the conductive path, or by using circuitry that is insensitive to the effects of conductive noise.
Since an actual fiber-optic communication network has many different types of circuitry, all operating at once, it is important that potential conducted noise problems be minimized to allow all the circuitry to operate without any section of it being adversely affected. In addition, most computing products have limits on how much conducted noise they are allowed to generate on the 120 Vac power lines to prevent them from disturbing other devices connected to the same AC power line.
17
Networking Technology
The demand for data and speed on the Internet has been increasing at an unprecedented rate. Avago Technologies satisfies this demand by producing fiber-optic components that transform electrical data into “packets network. This in turn helps Avago’s customers accelerate its evolution. Now, as never before, fiber is everywhere!
The optical network is composed of countless specialized networks. These networks enable groups of computers to communicate with each other, allowing users to share files and peripherals, send e-mail messages, and access remote servers.
The optical network provides a high-speed transmission path for the Internet and telecommunications networks. Avago’s fiber-optic components can be found in the equipment that is used in the following networks: x Enterprise (or Premise)--Local Area Networks provide connectivity within a building or campus.
x Metro/Access--Metropolitan Area Networks provide connectivity beyond the Enterprise, linking residential areas and LANs throughout a metropolitan area and providing access to Long-Haul Networks.
x Long Haul--Wide Area Networks provide connectivity for metropolitan networks throughout the world.
x SAN--Storage Area Networks link disk subsystems directly to one or more servers via a high-speed connection, providing high-volume data storage services.
Specialized equipment, many using Avago’s high quality, fiber-optic components, controls the flow of information across the optical network.
Table 5.
Equipment
Clients
Backbone
NIC
(Network Interface Card )
Server
Hub*
Switch
Switch/Router
Description
Computers that request to use files, send print jobs, and request other shared resources on the network. Client software (part of the network operating system) contains a redirector that captures requests from the PC application program (such as Microsoft
Word) and routes them out of the PC and across the network. (Also referred to as nodes or users.)
A high-speed, fiber-optic network that connects smaller LANs.
Card that connects computers or peripherals to the network via a cable. Inside the computer, NICs move data to and from the random access memory (RAM); outside the computer, NICs control the flow of data in and out of the network cable system. (Also known as LAN Adapter Cards.)
A computer or device on a network that manages network resources. For example, a file server is a computer and storage device dedicated to storing files. A print server is a computer that manages one or more printers; a network server is a computer that manages network traffic. A database server is a computer system that proc-esses database queries.
Stand-alone equipment with up to 24 physical interfaces that connect the nodes to the server. An active hub regenerates the signal using a repeater. Hubs function as junction boxes and can also be stacked. (Also called repeater or concentrator.)
A device in a network that filters and forwards packets between LAN segments. Switches operate at the data-link layer (layer 2) of the OSI (Open System Interconnection) Reference Model and therefore, support any packet protocol. LANs that use switches to join segments are called switched LANs or, in the case of Ethernet networks, switched Ethernet LANs.
Switch/Routers search inside the envelope surrounding the data to determine the data packet’s destination, read the information
(some routers even have error correction), and send the packet of information to the appropriate location. The Switch/Router at the receiving end repackages the data into a packet or frame appropriate for its LAN segment. A router may also function as a
“firewall,” by preventing unauthorized users from gaining access to the network.
DWDM Transmission
Equipment
Dense-Wavelength-Division Multiplexing combines numerous wavelengths of light (each one carrying different information) and sends them simultaneously along a single fiber-optic cable. The wavelengths are separated (demultiplexed) at the other end of the optical link.
SONET/SDH Transmission
Equipment
Large, complex optical equipment that transmits high-volume, high-speed data using SONET/SDH protocols from point to point in the Metro/Access and Long Haul Networks.
*An active hub or concentrator contains a management module that can answer queries from a management console.
18
An Enterprise (or premise) local-area network (LAN) is a system within a building or campus that connects the widely separated computer resources of a single organization. Within a building, each node (personal computer, workstation, server, or other equipment) attached to the LAN is able to share data with any other node on the network. As a result, workers can use the LAN to share devices, such as laser printers, send e-mail, and share files and applications. They can also share common connections outside the Enterprise, as for example, the Internet.
route the signals to the proper port so that they reach the intended destination.
Ethernet standards specify different distances and data rates for connections in the LAN. Avago sells a these applications. Small form factor dimensions allow a higher density of transceivers to be placed on a blade.
Figure 23.
Metro/Access Networks provide connectivity beyond
Figure 22.
Often, one large LAN (one with many nodes--users) may be segmented into smaller LAN subnets within or between buildings and connected by a Switch/Router.
In a building, a network is composed of various segments of different lengths, transmitting data at speeds determined by user demand. Avago sells components for equipment used in each of the various optical segments in the corporate LAN environment.
Figure 23.
A number of standards have been established for products that link different computers and network equipment from various vendors; the most common standard is Ethernet.
throughout a metropolitan area and provide access to
Long Haul Networks. In Metro/Access and Long Haul Networks, large complex optical systems transmit high-volume, high-speed data over fiber-optic cables.
Long Haul Networks link multiple Metro/Access networks, providing the ability to transmit data around the world. Compared to LAN data rates, which transmit at a maximum of 1 Gigabit per second, data rates in the Long Haul Network typically exceed 10 Gigabits per second. Voice and data from within Metro/Access Networks are combined at these higher rates to provide an economical transmission path between cities and continents.
As data moves from the Enterprise to the Metro/
Access and then to Long Haul Networks, it is continually combined and transmitted at higher data rates.
Common data rates in Metro/Access and Long Haul Networks include: x
155 Mbps (OC-3) x
622 Mbps (OC-12) x
2.5 Gbps (OC-48) x
10 Gbps (OC-192)
The higher data rates can be aggregates of multiple lower speeds. For example, four OC-48 equals one OC- 192.
These data rates are defined by SONET (Synchronous Optical Network), which is one of the main trans-
Avago’s fiber-optic components are an integral part of the blades (cards) that form Routers or Switches. Fiberoptic transceivers convert digital electrical signals to light pulses and light pulses to digital electrical signals for transmission on the optical link. The Switch Fabric inside the equipment manages the proper interconnections to
Haul networks. ATM (Asynchronous Transfer mode) is a common method for packaging data for transmission over SONET.
19
Within homes and businesses, the demand for exchanging increasing amounts of data with remote locations is driving the need for higher bandwidth in Metro/
Access Networks. Links close to the Enterprise and residential areas are typically 155 Mbps (OC-3) and are dominated by single-mode fiber connections. Avago is a leading supplier of single-mode, fiber-optic transceivers for these links.
Avago also provides single-mode, fiber-optic transceivers for SONET transmission within Metro/Access Networks. Links are typically 2.5 Gbps (OC-48).
Within the Long Haul network, DWDM (Dense Wave-
The primary value of this technology is the costeffective transmission of high-aggregate bit rates over long distances on a single fiber. Avago provides the fiber-optic transceivers for the links between the SONET terminals and DWDM transmission equipment, specifically for the line cards (blades) used in SONET transmission equipment.
The SONET standard specifies different distances and data rates for application in Metro/Access and Long Haul Networks. Avago provides a large variety of fiber- optic transceivers for OC-3, OC-12, and OC-48 applications. Smallform-factor transceivers are in these markets; their smaller dimensions allow a higher density of transceivers to be placed on a line card.
combine multiple SONET channels onto one fiber.
Note: white dots show where Avago’s components are used.
Figure 25.
20
Table 6. Avago Local Area Network Components
Standard
Ethernet
Fast
Ethernet
Gigabit
Ethernet
802.3
802.3u
802.3z
Speed
(Mbps)
10
100
1,000
Maximum
Segment Length
2 km MMF
2 km MMF
550 m MMF
10 km SMF
Product Types
Optical Transmitters
Optical Receivers
Standard 1x9 and 2x5 SFF MT-RJ SFF
Optical Transceivers
Standard 2x5 SFF MT-RJ & SFP Pluggable LC
Optical Transceivers
Optical GBICs
SerDes ICs
Table 7. Avago Local Area Network Components Features and Benefits
Features
High-volume, industry-standard, fiber-optic transceivers up to 1 Gbps
Benefits to Customers
Assurance of supply
Application engineering support
Reference Designs
Interoperability with supporting ICs
Reduced time to market
Ease of Design
High Reliability Reduces downtime for networking equipment end users
Continually introduce products with higher density and greater bandwidth Provides for continual evolution of the Internet
Complete product line of LAN standards and multiple
form factors, including fiber-optic transceivers and SerDes ICs
Simplifies supply chain
Table 8. Avago’s Metro/Access and Long Haul Components
Standard
SONET OC-3
OC-12
Speed (Mbps) Maximum Segment Length
SR IR LR
155
622
2 km
MMF
15 km
SMF
40 km
SMF
OC-48 2,500
OC-192 10,000 Proprietary
Network Products
Type
Metro/Access Standard & SFF
Metro/Access
Optical Transceivers
Long Haul
Long Being
Investigated
Footprints
1x9, SFF MT-RJ
1x9, SFF MT-RJ & LC
1x9, 2x9, SFF MT-RJ & LC
21
Table 9. Avago’s Metro/Access and Long Haul Components Features and Benefits
Features
High-volume, on 155 Mbps (OC-3), 622 Mbps (OC-12), and 2.5 Gbps (OC-48) industry- standard, fiber-optic transceivers
Benefits to Customers
Assurance of supply
Satisfies demand for single-mode, fiber-optic transceivers
Application engineering support Interoperability with supporting ICs
SONET compliance
Reference Designs
High Reliability
Reduced time to market
Ease of Design
Reduces downtime for networking equipment end users
Telecordia (formerly Bellcore) compliant
Continually introduce products with higher density and greater bandwidth Provides for continual evolution of the Internet
Multiple form-factor, fiber-optic transceivers for Metro/Access standards Simplifies supply chain
LAN Architecture Evolution
At first, all LANs were shared, allowing only one transmission at a time. In this arrangement, each device waits for access to the LAN before transmitting. Bandwidth is shared among the nodes.
As the number of nodes increases, the bandwidth for each node decreases. To alleviate the problem of shared
LANs, bridges and routers were created to segment traffic into smaller LANs. This permitted fewer devices to be connected to each hub, in effect, increasing the bandwidth on it.
When do you use a bridge and when do you use a router?
As shown in Figure 26, if two different networks are to be joined, use a router. If two similar networks are to be joined use a bridge.
(WKHUQHW
+XE 5RXWHU
7RNHQ
5LQJ
(WKHUQHW
+XE %ULGJH
+XE
(WKHUQHW
Figure 26.
22
The problem with lack of bandwidth led to the development of switches. A switch is basically a multi-port bridge.
Switched LANs allow a virtual dedicated point-to-point connection between multiple devices on the LAN, allowing access to full bandwidth for each connection
(similar to a phone system experiencing many simultaneous phone calls). With a switch, each node has access to the full bandwidth. Switches work best in a uniform traffic flow, for example, 10 to 20 desktop computers communicating with each other, as opposed to one device receiving most of the traffic.
In full duplex switching, each node has equal bandwidth both transmitting and receiving, which can be exercised simultaneously, effectively doubling the bandwidth requirement. The node can “talk” and “listen” at the same time as in a telephone call. In this situation, special NICs, hubs and a high through-put backplane are required.
Table 10 compares routers and switches.
Table 10.
Routers Support
- Software-based route determination and packet processing
Switches Support
- Hardware processing (provides higher throughput)
- Full-duplex switched links between NICs (reduces latency) node-address resolution
- Traffic isolation and filtering
- Network management
- Protocol conversion and mismatch resolution
Access Methods
Access methods determine how data shares a common network path.
Carrier Sense Multiple Access with Collision Detect (CSMA/CD)
In this method, a node’s NIC can continually sense the traffic on the network. When the node has a frame to send, the NIC checks to see if the network path is clear. If it is, it sends the data. If data “collides” because two nodes send at the same time, each node will sense it, stop sending and wait for a random time before trying to put its data on the network again. Collisions represent only about 1 to 10% of active time in networks. Equal access is guaranteed only on a probabalistic basis. Ethernet uses CSMA/CD.
Token Ring requires a “manager” or active monitor for the ring. The active nodes negotiate, using their serial numbers, to determine which node is the active monitor. The monitor initiates the first “free” token and does general ring operation maintenance. This method guarantees equal access to the network.
A comparison between CSMA/CD and token passing are shown in Table 11.
Tab le 11.
Uses
Light/moderate traffic
CSMA x
Token Passing
Consistent heavy traffic x
Token passing
Office Applications x
Token Ring networks and FDDI networks use token passing. A node can transmit on the network only when it has the token. The token travels around the ring and stops at each node to see if it has anything to send. When a node is ready to send data, it marks the token frame as busy, attaches its data and destination information, and passes the token on. The token continues to travel around the network until it reaches its destination. At this point the recipient removes the information and indicates on the token frame that the information has been received. The token returns to the originator where confirmation is acknowledged, the token is then released as “un-busy.”
Medical Imaging
Cheap, easy flexible
Demand Priority
x x
Monitoring x
Demand Priority is a deterministic access method similar to token ring passing. The hub successively scans the ports to determine which one is ready to transmit data. This avoids the collisions that can occur with CSMA/
CD.
23
LAN Standards
Ethernet
Overview
Ethernet, an established standard since the mid 1980s, is the most widely used local-area network (LAN) transport protocol in the world today. Table 12 summarizes its data rates (the structure and protocol are identical for all three).
Table 12.
Fast Ethernet
Gigabit Ethernet
Data Rate
100
1000 stable, well understood LAN standard; and network standards that emerged after Ethernet have not succeeded in replacing it. Standards such as Token Ring and FDDI have been adopted by some companies in niche markets, but have not acquired the same market share.
Ethernet’s ability to quickly adapt to faster signaling rates is one reason why it has remained popular. Because Ethernet is familiar to the standards committees, adjusting the standards to meet new data rate requirements is a
MBd lot easier than adopting a completely new standard. With
125
1250 the introduction of Fast Ethernet and the emergence of
Gigabit Ethernet, IEEE 802.3 is not likely to be replaced in the near future and is in a good position to remain the most-used LAN standard in the world.
Ethernet is a shared bus network with a CSMA/CD (Carrier-Sensing Multiple Access with Collision Detect) access scheme. The encoding scheme for 10 Mbps Ethernet is
Manchester; Fast Ethernet uses 4B/5B, and Gigabit Ethernet uses a 8B/10B encoding scheme.
Most Ethernet configurations today use a central Hub specified by the LAN standard. The ring architecture is hidden inside the Hub, and the LAN management is centrally located. The 10BaseT, 10BaseFB, and 10BaseFL versions of the Ethernet standard specify a Hub-based Ethernet structure.
3HUVRQDO
&RPSXWHU
:RUNVWDWLRQ
+XE
3HULSKHUDO
:RUNVWDWLRQ
As speeds continue to increase, the market has shifted from 10 Mbps Ethernet to Fast and Gigabit Ethernet and eventually to 10 Gbps. One way that equipment manufacturers are making this transition easier for their customers is by offering them 10/ 100 hubs, which have both
10 Mbps and 100 Mbps connections.
The main points to keep in mind when selling into Ethernet applications are x
Although 10 Mbps is still the majority of installed networks, it will be phased out over the next few years as
Fast and Gigabit Ethernet takes over.
x
Fast Ethernet is growing at a very high rate and is also the biggest network standard at that speed, which includes ATM and FDDI.
x
10/100 hubs are very popular today among OEMs.
x
Manufacturers are very interested in Gigabit Ethernet and will begin using it for backbone connections as soon as the standard is approved (customers are designing with it now).
x
Ethernet is a shared network, not a dedicated will be more in favor of the ATM standard, which can handle voice, video, and data simultaneously.
6HUYHU
Figure 27.
Ethernet today accounts for approximately 90% of the installed copper and fiber networking nodes. Most network designers prefer to work with Ethernet because it is a
24
Standards
Ethernet, whose base identification is IEEE 802.3, is a LAN standard of the IEEE. The tables below show the various standards and the major specifications that all Ethernet networks must follow.
Table 13. Ethernet (10 Mbps/20 MBd) IEEE 802.3
IEEE 802.3 Specification Other Terms Transmission Medium Signal Type Maximum Link Length
10BaseT
10BaseFB [1]
- - - - - - -
- - - - - - -
Twisted Wire Pair
Multi Mode Fiber
Electrical
820 nm Optical
10BaseFL(FA) [1]
10BaseFP [2]
- - - - - - -
Fiber Passive
Multi Mode Fiber
Multi Mode Fiber
820 nm Optical
820 nm Optical
FOIRL [3] - - - - - - Multi Mode Fiber 820 nm Optical
Notes:
1. Active - repeater used (more components)
2. Passive - repeater not used (fewer components)
3. Fiber Optic Inter-Repeater Link: a fiber-optic link that connects two separate Ethernet LANs.
Table 14. Fast Ethernet (100 Mbps/125 MBd) IEEE802.3u
IEEE 802.3u Specification Transmission Medium
100Base-T UTP*
100 Base-FB
100Base-FL
Multimode Fiber
Multimode Fiber
Signal Type
1300nm Optical
1300 nm Optical
100Base-FP Multimode Fiber
*Unshielded Twisted Pair
Note: For Ethernet and Fast Ethernet, there is no single-mode specification.
1300 nm Optical
Table 15. Gigabit Ethernet (1000 Mbps/1250 MBd) IEEE802.3z
Transmission Medium
UTP*
62.5/125 μm fiber
50/125 μm fiber
50/125 μm fiber
62.5/125 μm fiber
9/125 μm fiber
*Unshielded Twisted Pair
Signal Type
850nm optical
850nm optical
1300nm single-mode
1300nm single-mode
1300nm optical
Maximum Link Length
220/275 meters
500/550 meters
550 meters
550 meters
5 km
100 meters
2 km
2 km
500 meters
1 km
Maximum Link Length
2km
2 km
500 meters
25
The various layers in Figure 28 are described below and explanations of abbreviations used are given in Table 16.
Table 16.
Abbrev. Explanation
26,
5HIHUHQFH/D\HUV
$SSOLFDWLRQ
3UHVHQWDWLRQ
6HVVLRQ
7UDQVSRUW
1HWZRUN
'DWD/LQN
3K\VLFDO
8SSHU26,/D\HUV
/RJLF/LQN&RQWUROV//&
0,,
0HGLD$FFHVV&RQWURO0$&
5HFRQFLOLDWLRQ 5HFRQFLOLDWLRQ
*0,,
3&6
$8,
0',
30$
0HGLXP 0',
0EV0EV *EV
30$
30'
0HGLXP
Figure 28. IEEE 802.3 Ethernet Physical Layers vs. Data Rate OSI Layers
AUI
GMII
MAC
MDI
MII
OSI
PCS
Attachment Unit Interface
Gb/s Media Independent Interface
Media Access Control
Medium Dependent Interface
Media Independent Interface
Open System Interconnections
Physical Coding Sublayer
PMA
PMD
Physical Medium Attachment
Physical Medium Dependent
• Layers 5, 6, and 7 support inter-operability
• Network (3, IP) and transport (4, TCP) support
InterNetworking
• Physical (Level 1) and data link (Level 2) are specified for the LAN
PLS
TCP
Physical Layer Signaling
Transmission Control Protocol
Network (Level 3) Routers and Switches
• Utilize IP Protocol for routing and packet segmentation
• Packets are routed end-to-end using the Level-3 source and destination logical addresses located in the
IP header
• Packets are transported point-to-point between
Data Link (Level 2) Bridges and Switches
• Utilize MAC Addresses only and cannot scale to larger networks addresses
0EV6KDUHG
0EV
5HSHDWHU
0EV%DFNERQH
0EV1RGHV
/HYHO
6ZLWFK
*EV%DFNERQH
/HYHO6ZLWFK
0EV
1RGHV
/HYHO6ZLWFK
%ULGJHV /RFDO
5RXWHUV
0EV
/HYHO
5HSHDWHU
$OO0EV1RGHV
0EV
1RGHV
0EV/LQNV
0EV/LQNV
:$1
5RXWHU
3XEOLF
1HWZRUN
0EV
/HYHO6ZLWFK
:$15RXWHU
6HUYHU 6HUYHU
Figure 29. Ethernet LAN Collapsed Backbone Network Evolution
0EV
1RGHV
3XEOLF
1HWZRUN
6HUYHU
*EV/LQNV
*EV
/HYHO6ZLWFK
:$15RXWHU
6HUYHU
26
:RUG
*0,, 3&6 30$ 30' 0',
73 73
E 3
%\WH
E
&KDUDFWHU
EE
(QFRGHU
6
ELW
6HU'HV
%LW
7[
2SWLFDO
)LEHURU
&RD[LDO
&DEOH
73 73
6 E
5[
3 E
:RUG
ELW
6HU'HV
%\WH &KDUDFWHU
'HFRGHU
Figure 30. Gb/s Ethernet (GbE) Physical Link Interfaces
%LW
The Ethernet Link
The structure of an Ethernet link depends on the transmission medium. As shown in Figure 31, the Ethernet Controller chip is common to all Ethernet adapter cards; and depending on the network speeds, it has different functions.
(WKHUQHW&RQWUROOHU
73,
$8,
873RU
&RD[LDO
6\VWHP
%XV
(WKHUQHW&RQWUROOHU
)LEHU2SWLF,QWHUIDFH&DUG
$8,
%DVH)/
6LQJOH&KLS
7UDQVFHLYHU
+3
7UDQVPLWWHU
+3
5HFHLYHU
)LEHU2SWLF0$8
00)
60)
Figure 31. 10 Mbps Ethernet
The gray box is the fiber-optic interface card; above it is the corresponding copper interface. The functions of the interface card component’s are described in Table 17.
Table 17.
Block Description
Ethernet Controller Chip [1] Performs all functions concerning bus interfacing, buffering/framing, multi-plexing/demultiplexing, encoding/decoding, and clock and data recovery [2] .
TP Interface
AU Interface
Interfaces directly to the twisted-pair wire cable.
Interfaces directly to the coaxial cable or the fiber optic MAU.
10Base-FL Single-Chip
Tranceiver
Transmitter
Converts data from the AUI into format required by the optical transmitter and receiver. Contains the drive and receive
(quantizing) circuitry. Also monitors functions for transmission, reception, collision and error.
Contains the LED or ELED.
Receiver Contains the pin photodiode and transimpedance amplifier.
Notes:
1. Available from several IC vendors.
2. Clock and data recovery is included in most Ethernet Controller Chips.
27
(WKHUQHW&RQWUROOHU
$8,
73,
$8,
2SWLFDO7UDQVFHLYHU
7UDQVFHLYHU
873
6\VWHP
%XV
(WKHUQHW&RQWUROOHU
00)
60)
)LEHU2SWLF,QWHUIDFH&DUG
Figure 32. 100 Mbps Fast Ethernet
Table 18.
Block Description
Ethernet Controller Chip Performs all functions regarding bus interfacing, buffering/framing, multiplexing/demultiplexing, encoding/decoding, and clock and data recovery.
TP Interface
AU Interface
Optical Transceiver
Interfaces directly to the twisted-pair wire cable.
Interfaces directly to the fiber optic transceiver.
Includes LED or laser, PIN/preamp, drive, and quantizing circuitry.
(WKHUQHW&RQWUROOHU 6(5'(6 873
6\VWHP
%XV (WKHUQHW&RQWUROOHU
6LQJOH&KLS
7UDQVFHLYHU
2SWLFDO
7UDQVFHLYHU
)LEHU2SWLF,QWHUIDFH&DUG
Figure 33. 1000 Mbps Gigabit Ethernet
Table 19.
Block Description
Ethernet Controller Chip Includes bus interfacing, buffering/framing, encoding/decoding, and clock and data recovery.
SERDES chip
Optical Transceiver
Interfaces to the fiber-optic transceiver or directly to the UTP, includes serializing/deserializing function.
Includes VCSEL or Fabry-Perot laser emitter, PIN/preamp, drive and quantizing circuitry.
00)
60)
28
Gigabit Ethernet
As new and existing network applications evolve to embrace high-resolution graphics and highvolume data,
Full- and Half-Duplex over Fiber Today, UTP in the Future
demand is increasing at the desktop, the server, the hub, and the switch for increased bandwidth. The simple migration and support offered by Ethernet, combined with the scalability and flexibility to handle new applications and data types, makes Gigabit Ethernet the strategic choice for high-speed, high-bandwidth networking.
Gigabit Ethernet supports new full-duplex operating modes for switch-to-switch and switch-to-endstation connections. Initially operating over optical fiber,
Gigabit Ethernet will also be able to use Category 5 UTP
(Unshielded Twisted Pair) cabling.
(WKHUQHW8SSHU/D\HUV
0HGLD$FFHVV&RQWURO0DF
IXOOGXSOH[DQGRUKDOIGXSOH[ Gigabit Ethernet is an extension of the highly successful 10 Mbps and 100 Mbps IEEE 802.3 Ethernet standards.
It offers a raw data bandwidth of 1000 Mbps, while maintaining full compatibility with the huge installed base of
Ethernet nodes.
*LJDELW0HGLD,QGHSHQGHQW,QWHUIDFH*0,,RSWLRQDO
%%
HQFRGLQJGHFRGLQJ
%DVH7
HQFRGHUGHFRGHU
The Gigabit Ethernet standard has the following attributes:
• allows half- and full-duplex operation at speeds of 1000 Mbps
%DVH/;
/:/
)LEHU2SWLF
7UDQVFHLYHU
60)NP
X00)P
X00)P
%DVH6;
6:/
)LEHU2SWLF
7UDQVFHLYHU
X00)P
X00)P
%DVH&;
6KLHOGHG%DODQFHG
&RSSHU
7UDQVFHLYHU
P
%DVH7
873
&DWHJRU\
7UDQVFHLYHU
P
]3K\VLFDO/D\HU
DE
3K\VLFDO/D\HU
• uses the 802.3 Ethernet frame format
• uses the CSMA/CD access method with support for one repeater per collision domain
• addresses backward compatibility with 10Base-T and
100Base-T technologies
Distance Specifications for Fiber Optic Media
As the bandwidth of the fiber increases, the minimum range for multi-mode fiber increases up to 550 meters. The longwave length transceiver (1000Base-L X ) reaches 550 meters for all media types. For single-mode fiber with 1000Base-L X , the distance is specified at 5 km.
The link distance for the lowest bandwidth multi-mode fiber was specified at 220 meters. Other fiber types can go farther.
Three features of the Gigabit Ethernet link distances are:
1. a multimode fiber-optic link with a maximum length of 550 meters
Figure 34.
Gigabit Ethernet adheres to a physical-layer standard. In general, the PHY or physical layer is responsible for defining the electrical and procedural characteristics for establishing, maintaining and deactivating the physical link between network devices. Two PHYs provide Gigabit transmission X is targeted at lowest-cost multi-mode fiber runs in horizontal and shorter backbone applications. 1000BASEL X is targeted at longer multi-mode building fiber backbones fiber, these standards define gigabit transmission over maximum distances ranging from 220 m to 550 m. Single-mode fiber, which is covered by the long-wavelength standard, is defined to transmit up to 5 km; some transceivers, including Avago’s, are defined to transmit up to 10 km.
2. a single-mode fiber-optic link with a maximum length of 10 kilometers
3. a copper-based link with a maximum length of at least
25 meters
29
Gigabit Ethernet Products
Because Gigabit Ethernet is Ethernet, Gigabit Ethernet product types are quite straightforward: Layer-2 switches,
Layer-3 switches (or routing switches), uplink/downlink modules, NICs, Gigabit Ethernet Router interfaces, and buffered distributors.
There are pure multi-port Gigabit Ethernet switches with high-performance backplanes, as well as devices that have both Gigabit Ethernet and Fast Ethernet ports in the same box. Gigabit Ethernet uplinks have appeared as modular upgrades for fixed-configuration Fast Ethernet devices or modular, chassis-based hubs to provide a highspeed connection to the network.
Figure 35.
30
Token Ring
Overview
Token Ring (IEEE 802.5), originally developed by IBM, has two versions: 4 Mbps and 16 Mbps. The faster version is generally used as a backbone.
Topology
Token Ring access was discussed in the Networking Section. In a Token Ring LAN, the devices (nodes) are connected in a ring that forms a shared LAN; information is passed in only one direction.
The token-passing scheme for LAN allocation deterministically ensures that each device has equal access to the LAN over both short and long intervals of time. In comparison, equal access to an Ethernet LAN is ensured on a probabalistic basis only over long periods of time.
In cases where guaranteed timely access to the LAN is needed, Token Ring is preferred.
Token Ring also has more complex LAN management, which provides additional services for the LAN, including information on routing and connection problems. This additional complexity, however, results in a slightly higher cost per node.
Structure
Most installations today consist of a central Token manages the LAN. The Token Ring LAN does not appear to be a ring because the wiring is hidden inside the hub.
wiring for the LAN is in the form of a “star” network, as shown in Figure 36.
The Token Ring standard specifies a hub-based wiring structure. The hub, which is centrally located,
3HUVRQDO
&RPSXWHU
The structure of a Token Ring link depends on the transmission medium. As shown in Figure 37, below, the Token
Ring controller chip is common to all Token Ring adapter cards. The serial transmit and receive circuitry and media interface depends on which transmission medium is used. This circuitry must be composed of discrete components. These components are described in Table 20.
Table 20.
6HUYHU
:RUNVWDWLRQ 3HULSKHUDO
Block Description
Token Ring
Controller
Chip
Bus Interface, Multiplexing/Demultiplexing, Encoding/
Decoding, Buffer-ing/Framing, Clock and Data Recovery*
TP Interface Interfaces directly to the twisted-pair wire cable.
AU Interface Interfaces directly to the coaxial cable or the fiber optic
MAU
Transmitter HFBR-1414Z - Contains the LED
Receiver HFBR-2416Z - Contains the pin photodiode and transimpedance amplifier.
*Available from several IC vendors.
Figure 36.
7RNHQ5LQJ
+XE
7RNHQ5LQJ&RQWUROOHU
73,
$8,
873RU
&RD[LDO
6\VWHP
%XV
7RNHQ5LQJ&RQWUROOHU
$8,
/('
'ULYH
&LUFXLWU \
5HFHLYH
&LUFXLWU \
7UDQVPLWWHU
5HFHLYHU
)LEHU2SWLF0$8
00)
60)
)LEHU2SWLF,QWHUIDFH&DUG
Figure 37. Token Ring Media Access Unit
31
Fiber Distributed Data Interface
Fiber Distributed Data Interface (FDDI) Network Overview
Fiber Distributed Data Interface (ANSI oped in the ANSI Standards Committee throughout the
1980’s, is a Local Area Network standard designed for transmission over both single- mode and multi-mode fiber-optic cable. “Backbone” applications use this LAN standard because it provides 100 Mb/s bandwidth (Ethernet runs at 10 Mb/s and Token Ring runs at 16 Mb/s).
Topology
X 3T9.5), devel-
As shown in Figure 38, FDDI is a redundant-ring, tokentions between groups of slower network devices. The
FDDI “back-bone” refers to the central dualring structure.
The outer ring is usually referred to as A and the inner ring as B. Only the A ring is used for primary network transmission, and the information (as with Token Ring) is transmitted on this ring in only one direction. The second, or redundant ring, B, transmits information in the opposite direction and is used for fault tolerance.
Should the primary ring develop a discontinuity due to a break in the fiber cable or a device shutting down, the secondary ring is activated. The network then detours the transmission onto the B ring avoiding the discontinuity.
As shown in Figure 39, this scheme provides fault tolerance by maintaining an intact ring structure.
0DLQIUDPHRU
6XSHUFRPSXWHU
:RUNVWDWLRQ
&RQFHQWUDWRU
&RQFHQWUDWRU
:RUNVWDWLRQ
:RUNVWDWLRQ
)'',
0EV
:RUNVWDWLRQ
:RUNVWDWLRQ
:RUNVWDWLRQ
6HUYHU
Figure 38. Typical FDDI Network
%UHDNLQ&DEOH
Figure 39.
Protocol
As with the Token Ring LAN, FDDI allocates access to the
LAN using the Token Passing method. For a description of how this LAN allocation scheme works, please refer to the
Networking section.
numerous nodes (or desktops) to the network. As Figure
40 shows, the number of connections to the desktop is much greater than the number of connections to the
FDDI backbone.
As with Ethernet and Token Ring, FDDI is a shared LAN. The total bandwidth of the LAN is equally divided between the devices connected to it. The more devices that are added, the less bandwidth each device gets.
Most networks today use concentrators, which connect multiple devices to the central FDDI backbone.
It is possible to “stack” these concentrators to connect
Standards
The MIC (Media Interface Connector) standard was defined specifically for use in the FDDI network.
To decrease the possibility of simple wiring errors (for example, connecting the A-ring cable into the B-ring transceiver of the box) the standards committee designed special keys for each connector/port mating.
32
&RQFHQWUDWRU
:RUNVWDWLRQ
&RQFHQWUDWRU
:RUNVWDWLRQ
:RUNVWDWLRQ
%5LQJ
$5LQJ
:RUNVWDWLRQ
:RUNVWDWLRQ :RUNVWDWLRQ
Figure 40.
Encoding Scheme
An FDDI LAN uses a 4B/5B encoding scheme that is
80 percent efficient. This means that for every 4 bits of raw (useful) data being sent, 5 signal elements are
Table 21.
a serial signal rate of 125 MBd to transmit 100 Mb/s.
The resulting serial signal for an FDDI link is 125 MBd.
Key
Type Location Purpose
A Any device connected to the backbone Connect to A ring
B Any device connected to the backbone Connect to B ring
M Non-backbone side of a concentrator Connect to end device
S End Device connected to the concentrator Connect to concentrator
Other connector styles accepted by the standards comof AT&T) connector and the SC connector, shown in
Figure 42.
.H\
Figure 41.
67
Figure 42.
67
The keying options are based on the specific part of the FDDI LAN that the data communication link fits.
The link from a concentrator to a device was defined as a “master-slave” link, while the two redundant rings
(described earlier) were called the A ring and B ring.
The keying options, shown in Table 21, were then defined.
CDDI (Copper Distributed Data Interface, which is FDDI over twisted-wire pair) has emerged as low-cost technology link with a maximum length of 100 meters. The backbone of an FDDI network, which has a maximum link length of 2 km between devices, will continue to use fiber optics.
33
%0$&6\VWHP
,QWHUIDFH%6,
%DVLF0HGLD
$FFHVV&RQWURO
%0$&
%XIIHULQJ
)UDPLQJ
%%
(QFRGH
'HFRGH
0XOWLSOH[RU
'HPXOWLSOH[RU
15=,15=,
(QFRGH
'HFRGH
&ORFNDQG'DWD
5HFRYHU\
&RPSRQHQWV
/('
'ULYHU
4XDQWL]HU 3UHDPS
30'/D\HU
7[
5[
)LEHU
2SWLF
&DEOH
0$&/D\HU 3+</D\HU
Figure 43. FDDI Adapter Card
FDDI Structure
The standard FDDI link is composed of four distinct layers: the MAC (Media Access Control) layer, the PHY (Physical) layer, the PMD (Physical Media Dependant) layer, and
SMT (Station Management). These layers are described in the following.
Smaller footprint (1 row of 13 pins) were offered at a lower price. As time passed, this pin configuration became more popular. Then, even smaller products (1 row of 9 pins, with a dual SC connector) were introduced to compete for these PMD low-cost fiber design-ins.
(Products compliant with the FDDI LCF PMD standard, which has less stringent specifications than the original
PMD standard, can be low-cost options for fiber-optic data links.) These products are available in both multimode and single-mode versions. Customers of FDDI MIC transceivers are using the 1 X 9 foot print products due to limited board space and lower cost.
;
Figure 44.
;
;
Table 22.
Layer Description
MAC (Media Access Control) Controls flow of signals to and from the bus interface.
PHY (Physical Layer) Performs all functions related to buffering/framing, multiplexing/demultiplexing, encoding/decoding, clock and data recovery.
PMD (Physical Media Dependant) Controls the transmitter drive circuitry, transmitter (1300nm LED or Laser), receiver (1300nm PIN photodiode), receive circuitry (quantizer).
SMT (Station Management) Manages the configuration of the network and reconfigures it if a discontinuity occurs, for example, a break in the fiber or a device shutting down.
34
ATM (Asynchronous Transfer Mode)/SONET Network
Overview
ATM is a network technology that provides voice, video to happen, the user needs a dedicated (uninter-
The switch establishes a virtual connection between a device and its requested counterpart, unless the requested counterpart is already part of another data
To provide these “dedicated” connections, the ATM standards committee chose a “Switched Network” in Figure
45. This arrangement allows multiple users to have full access to the bandwidth of the network without having to wait until the network is available.
network: if you dial someone’s number, you are given a dedicated link for your conversation, provided that the person you are calling is not already on the phone. The
ATM switch then routes the “call” to its proper address.
0DLQIUDPHRU
6XSHUFRPSXWHU
6WRUDJH
'HYLFH 6HUYHU
:RUNVWDWLRQ
3ULYDWH1HWZRUN
3ULYDWH
$70
6ZLWFK
:RUNVWDWLRQ
3HULSKHUDO
3ULYDWH
$70
6ZLWFK
3ULYDWH
$70
6ZLWFK
7RNHQ5LQJ
/$1
0XOWL
3URWRFRO
5RXWHU
(WKHUQHW/$1
)'',/$1
:$1
3XEOLF
$70
6ZLWFK
3XEOLF1HWZRUN
Figure 45.
Table 23. ATM / SONET End-User Products
Product Description
ATM Switch At the center of the ATM network, it directs the requests and establishes the virtual, dedicated connec-tions between the two distinct devices (nodes). Connected to the switch are all the separate units that are tied into the network. Each unit is connected to the switch via a bi-directional data-communication link, based on the SONET standard.
ATM Switches are of two different kinds:
1. The Private ATM Switch: For interconnecting multiple ATM Switches in the Private Network, as well as connecting to the desktop.
2. The Public ATM Switch: For interconnecting multiple private ATM switches, as well as multiple public ATM switches. It is expected that there will be a network of public switches in the WAN.
ATM Adapter Card Inserted into a workstation or PC to connect it to the ATM Switch, and hence into the ATM Network. Each adapter card has a duplex
ATM Interface link. The next page shows a simple block diagram of an Adapter Card.
Multi-Protocol Router An important part of the first implemented ATM Networks. It allows users on existing LANs access to the ATM Network. It forms the
“bridge” between the two LAN standards and maps the data from one format to another format.
The ATM Standard and Architecture Model
As shown in Figure 46, the ATM standard separates its links into two catagories: UNI (User-to-Network Interface) and NNI (Network-to-Network Interface). The majority of Avago FCD products are used in private UNI links.
&3(
&3(
81,
81,
$70
6ZLWFK
$70
6ZLWFK
$70
6ZLWFK
81,
&3(
81, 8VHUWR1HWZRUN,QWHUIDFH
11, 1HWZRUNWR1HWZRUN,QWHUIDFH
&3( &RQVXPHU3UHPLVHV(TXLSPHQW
Figure 46. Public B-ISDN Network
35
SONET Protocol for ATM Transmission
Instead of inventing a new high-speed, point-tostandard is SDH (Synchronous Digital Hierarchy). Occasionally, the two are used together: SONET/SDH. The ATM architecture is above the SONET physical layer specifications. Avago designs its optical products to conform to SONET specifications.
The choice of SONET as the data communication structure allows:
1. An already proven and understood technology for the high-speed datacom links. SONET link speeds are scalable: 155 Mb/s, 622 Mb/s, 2.488 Gb/s.
telecom standard.
The SONET standard specifies a 100 percent efficient technique for encoding the date: for example, the serial rate for a 155 MBd link is 155 Mb/s.
The Avago components shown in Figure 47, is the illustration of an ATM/SONET implementation are described in Table 25. Avago FCD supplies products for the SONET/
PMD Interface block of an ATM link. The particular Avago product depends on the portion of the ATM standard that the user’s product addresses (see the previous table for a description of physical-layer specifications).
Table 24. SONET Physical Layer Specifications for 1300 nm MLM Lasers
Specification
Data Rate
Distance
OC-3 OC-3 OC-3 OC-12 OC-12 OC-12
SR IR LR SR IR LR
155 Mb/s
0 - 2 km
155 Mb/s
0 - 15 km
155 Mb/s
15 - 40 km
622 Mb/s
0 - 2 km
622 Mb/s
0 - 15 km
622 Mb/s
15 - 40 km
Specification
Data Rate
Distance
OC-48 OC-48 OC-48 OC-192
SR IR LR SR
2.488 Gb/s
0 - 2 km
2.488 Gb/s
0 - 15 km
2.488 Gb/s
15 - 40 km ?
9.95-10.5 Gb/s
2km
6\VWHP
%XV
$70
%XV
,QWHUIDFH
DQG
&RQWURO
6HJPHQWDWLRQ
DQG
5HDVVHPEO\
621(7$70
81,3URFHVVRU
&ORFN
DQG
'DWD
5HFRYHU\
/('
'ULYHU
4XDQWL]HU
&RPSRQHQWV
3UHDPSOLILHU
)LEHU
2SWLF
&DEOH
Figure 47. ATM/SONET block diagram
Table 25.
Block Description
ATM Bus Interface and Control Responsible for the bus interface.
Segmentation and Reassembly (SAR) Frames the transmitted data, multiplexes voice, video, and data.
SONET/ATM UNI Processor Converts the data from the ATM format into the SONET format. It is responsible for the encode/decode and Mux/Demux functions
SONET/PMD Interface The physical media dependant part of the link. On the transmit side it involves: - the drive circuitry - the transmitter
(laser or LED) And on the receive side it involves: - the receiver (pin photodiode) - the receive circuitry (amplifier/ quantizer stage) - the clock and data recovery (CDR) circuitry
36
Fiber Channel
Fiber Channel Overview
Fiber Channel was developed as a high-speed interconnection to serve the needs of both channels and networks. A channel provides direct or switched point-topoint connections between devices, typically between a processor and a peripheral device, with minimal delay, and error correction in hardware. Networks, on the other hand, are more software intensive with more overhead because they provide a more extensive range of tasks, including managing unanticipated connections and commands. To meet the needs for both networking and channels, the Fiber Channel standard has defined three Fiber
Channel topologies and three classes of service.
Fiber Channel Topologies
The three topologies of Fiber Channel are fabric (also connect), and Arbitrated Loop (FC-AL).
3RLQWWR3RLQW
:RUNVWDWLRQ
3HUVRQDO
&RPSXWHU
)LEUH&KDQQHO
)DEULF
3HULSKHUDO
6HUYHU
'LVN
$UUD\
0DLQIUDPHRU6XSHUFRPSXWHU
Figure 49.
Point-to-point Fiber Channel data communication links are used today for efficient transmission of large blocks of continuous time-sensitive data and are ideally suited for mass storage applications.
Arbitrated Loop, the third Fiber Channel topology used today, defines a method of connecting three or more devices without the use of a fabric. This architecture allows several devices to be connected in a ring, forming a “virtual single device,” as shown in Figure 50.
$UELWUDWHG
/RRS
6ZLWFKHG)DEULF
Figure 48.
Switched-fabric topologies are used today for clusterfabric can be a single switch or a network of interconnected switches, as shown in Figure 49.
'HYLFH
$UELWUDWHG
/RRS
3RUW
$UELWUDWHG
/RRS
3RUW
'HYLFH
$UELWUDWHG
/RRS
Figure 50. Mass Storage
'HYLFH
$UELWUDWHG
/RRS
3RUW
$UELWUDWHG
/RRS
3RUW
'HYLFHQ
)LEUH&KDQQHO
3RUW
)LEUH
&KDQQHO
'HYLFH
Fiber Channel Classes of Service
To accommodate the wide range of communicathree classes of service: between two devices only, and ensures continuous, uninterrupted connections for rapid data transfer. It provides guaranteed delivery, with an acknowledgment of receipt.
- Class 2 connection is a frame-switched service without a dedicated connection. Frame switching requires the switching mechanism to read the frame header information, then decide to route the payload to its destination. As with Class 1, it provides guaranteed delivery with an acknowledgment of receipt.
- Class 3 is a connectionless service that allows data to be sent rapidly to multiple devices at-tached to a fabric. With Class 3 service, there is no acknowledgement of receipt. It is sometimes referred to as a
“datagram” transfer.
37
Fiber Channel Efficiency
In October of 1989, the standards committee adopted data transmission via optical fiber.
Fiber Channel Standard
Fiber Channel is the general name of an integrated set of standards being developed by the American National Standards Institute (ANSI) X 3T11 committee.
Fiber Channel can be specified with different speed links, as shown in Table 26.
Included in this family of standards are the FC-PH ( X 3.230-
1994), Fiber Channel Physical standard, and the FC-AL
( X 3.272-199x), Fiber Channel Arbitrated Loop standard.
Table 26.
The FC-PH standard consists of the three lower
Fiber Channel Parallel Data Rate Serial Data Serial Signal levels, FC-0, FC-1 and FC-2 of the segmented standard
Speed (Mbytes/sec) Rate (Mb/s) Rate (MBd) datacommunication link defined in Fiber Channel (see
Figure 51).
Eighth 12.5 100 133
Fiber Channel Media
Quarter 25 200 266
The physical media defined in FC-0 are:
Half 50 400 531
Full 100 800 1062.5
• Optical Laser, LED
• Copper Coaxial, Twisted Pair
Double 200 1600 2125
Fiber Channel uses a special low-cost duplex SC connec-
Quad 400 3200 4250 tor for optical fiber interfaces; and a 9-pin, D-type connector (DB-9) for shielded twisted-pair (STP) copper interface.
A BNC transmitter connector and a TNC receiver connector are specified in the standard for coaxial connections.
1 H W Z R U N V
)& ,3, 6&6,
& K D Q Q H VO
+,33, 6%&&6 ,3 $70
)& &RPPRQ6HUYLFHV
)&
)&3+
)&
)UDPLQJ3URWRFRO)ORZ&RQWURO
(QFRGH'HFRGH
)&
0ELWVV 0ELWVV 0ELWVV 0ELWVV 0ELWVV 0ELWVV
Figure 51.
Table 27. Fiber Channel Link Description
Layer Function Description
FC-4 Bus Interface Maps the data from the bus format to the Fiber Channel format.
FC-3 Common Services Undefined in the standard and by-passed in current implementations.
FC-2
FC-1
FC-0
Signalling Protocol
Transmission Protocol
Physical Layer
Buffers and frames the data before transmitting it.
Encodes and decodes, using the 8B/10B encoding scheme.
Serialize/Deserialize, Clock and Data Recovery, PMD (Physical Media De-pendent) Interface
(dependent on the medium).
38
The Fiber Channel standards committee has segmented the standard data communication link into five distinct blocks, FC-0 through FC-4, as shown in Figure 52.
Avago (FCD and ICBD) has the broadest product offering in the Fiber Channel industry today. In addition to the breadth of FC-0 physical layer products of optical modules, Serialize/Deserialize (SerDes) ICs and
Gigabit Link Modules (GLM’s), Avago is also a recognized leader for protocol chips.
Physical Media Dependent (PMD) products are designed to interface with either copper or multi-mode
Channel hubs, switches, or mass storage devices. OEMs can also use some of these products as part of a workstation subassembly.
6HULDOL]HU
'HVHULDOL]HU
6\VWHP
%XV
)& )& )& )&
'ULYHU
&LUFXLWU\
7;
&ORFN
DQG'DWD
5HFRYHU\
)&
5HFHLYHU
&LUFXLWU\
30'
5;
7UDQVPLVVLRQ
0HGLD
00)60)
7:3&RD[
Figure 52. Fiber Channel Adapter Card
:RUNVWDWLRQ
)LEUH
&KDQQHO
)DEULF
6XSHUFRPSXWHU
:RUNVWDWLRQ
:RUNVWDWLRQ
:RUNVWDWLRQ
3RLQWWR3RLQW
Figure 53. Fiber Channel Topologies
)LEUH
&KDQQHO
$UELWUDWHG
/RRS
:RUNVWDWLRQ
)&6ZLWFK
)DEULF
)&6ZLWFK
)&6ZLWFK
3&
2WKHU/$1V
IRUH[DPSOH
$70DQG
(WKHUQHW
Figure 54. Fiber Channel Applications
6XSHUFRPSXWHU
)LOH6HUYHU
)&
3RUW
)&
3RUW
/$1
3RUW
)&
3RUW
GLVN
GULYH
GLVN
GULYH
GLVN
GULYH
GLVN
GULYH
'LVN$UUD\
)&
3RUW
GLVN
GULYH
GLVN
GULYH
GLVN
GULYH
GLVN
GULYH
)&
3RUW
39
Optical Transmission and Types of Fiber
Optical Transmission
The basis for optical transmission in a fiber is governed by
Snell’s Law. It states that at the boundary between two media, light bends toward the normal (a line perpendicular to the surface) when it enters the optically denser medium, and bends away from the normal as it enters the optically less dense medium.
Each medium has a value called its refractive index. It is expressed as the speed of light in a vacuum divided by the speed of the light in that medium. It is given by the following equation:
&ODGGLQJQ
&RUHQ
&ODGGLQJQ
Index of Refra c tion (n) =
Ve l o c ity of Light in a Va c uum
Ve l o c ity of Light in a Medium
> 1
Note: the index of refraction, n, of any material is never less than one.
Figure 57.
The refractive indices of a fiber’s core and cladding determine its numerical aperture, NA, a term that defines the angle of the largest off-axis ray that a fiber can transmit.
A fiber with a high NA can collect more light than a fiber with a low NA. The equation for numerical aperture is:
NA = n
2
1
n
2
2
A ray of light traveling across the boundary between two different optical materials may be reflected or transmitted, depending on the relative indices of refraction of the materials. A ray in the low-index medium, n
2
, always penetrates (is transmitted into) the high-index medium n
1
.
A ray (Figure 55) in the high-index medium, n
1
, can penetrate into the low-index medium, n
2
, ( n
1
> n the critical angle, there is a partial reflection.
2
) only if it is incident at less than a specific angle (the critical angle) for those materials. If the angle of incidence is less than critical angle, reflection is total and no fraction of the light penetrates into the second medium.
As an example, if n
1
= 1.22 and n
2
NA =
NA = 0.3
1
( 1 .22)
2
( 1 .
1 8)
2
= 1.18, then the numerical aperture is calculated as shown below:
Rays within the core that are incident on the inner wall of the cladding at an angle greater than the critical angle will be totally reflected. They will then strike the opposite wall at the same angle and again be totally reflected. This process continues all the way to the end of the fiber. This phenomenon is known as total internal reflection (TIR), a key principle in fiber optics. (The only loss of light is from scattering by foreign matter or by absorption).
e
F
Q e
F
Q
1RUPDO
Q
Q Q
Q
Figure 55.
Figure 56.
Optical fiber construction takes advantage of Snell’s Law in the following manner. The fiber consists of a core with a refractive index n
1
and a cladding with a refractive index n
2
. For the light to propagate through the fiber core, the refractive index of the core must be greater than the refractive index of the cladding, that is, ( n
1
> n
2
).
Figure 58.
Typically, light rays enter the fiber core within a range of angles relative to the fiber axis. The maximum angle at which an entering ray experiences TIR is the acceptance angle, θ
A
.
40
This angle, θ
A
, is related to the critical angle, which is determined by the refractive indices of the core and the cladding. The smaller the critical angle, the larger the acceptance angle, so light rays at larger angles are propagated along the fiber, as shown in Figure 60.
When the acceptance angle is rotated 360º around the fiber axis it forms an acceptance cone, as shown at the right. The larger the angle of the acceptance cone, the larger the NA. The larger the NA, the more light that the fiber can collect and transmit.
Light rays outside the acceptance cone strike the boundary between the fiber and the cladding at an angle less than the critical angle. As shown in the enlarged view in
Figure 61, these rays are not reflected along the fiber but are absorbed by the cladding and lost. The angles that the light makes are exaggerated here to make the illustration clearer.
$FFHSWDQFH
$QJOH
$FFHSWDQFH
&RQH
Figure 59.
e
$ e
$ e
F
Figure 60.
e
$ e
F e
F
Figure 61.
41
Types of Fiber
A number of fibers types are common in today’s marketplace.
Glass Fiber
Glass fiber is the most common type of fiber available, with companies such as Corning and Siecor producing billions of meters per year. Common industry-standard glass fibers are differentiated by the diameter of the core: larger cores allow more modes to propagate; smaller cores allow fewer. Glass fiber is available in three common sizes: two multimode fibers and one single mode fiber. Multi-mode fibers have larger cores and allow many modes to travel through the fiber. The two standard sizes for multi-mode fiber are 62.5/125 μm, and 50/125 μm.
The first number indicates the core diameter and the second number indicates the cladding diameter. Singlemode fibers have cores so small that only one mode of light enters the fiber. Most single-mode fibers are 9/125
μm, but some have 8 μm cores.
Glass fibers are lightweight, durable, and fairly inexpensive. They make up most of the installed base of fiber-optics in the world. Single-mode fiber accounts for all of the longhaul telecommunications fiber.
Plastic Optical Fiber (POF)
Plastic fiber has been around for over 20 years and makes up a small percentage of the total fiber in the world today.
Most plastic fiber is used in Japan and Europe.
POF is easy to work with because of its large 980/1000 μm diameter. As a result, component, connector, and labor costs are all minimal, making a plastic fiber-optic link comparable to the cost of copper wire.
Hard Clad Silica (HCS)
HCS fiber has a glass core and a plastic cladding, giving it the qualities of both materials. This fiber is used even less than plastic fiber. HCS is the most durable of fibers and is relatively easy to work with. It has a 200/240 μm diameter, and is available in riser and plenum versions that meet environmental standards.
If the diameter of the fiber core is smaller than the light emitter or larger than the photodiode detector, power coupled from the transmitter into the fiber or power coupled from the fiber into the receiver can be lost. For this reason, the transmitter and receiver must be optically matched to the fiber’s diameter.
42
Understanding a Fiber Optic Data Sheet
Introduction
The quickest way to locate the proper fiber-optic components for your customers is to first determine the requirement of their designs. You can assist them in identifying which specifications are most critical by reviewing the following items with them.
Data Sheet
As the name implies, this document identifies, describes and specifies the performance of a particular link or its components under specific operating conditions.
Package dimensions are specified also. Major features of the product and the advantages that these features yield are listed; the benefits derived from these features are explained. This information helps customers to properly design, which helps them to apply the link or the various components in the best way.
Key Specifications
1. Signaling Rate (Link)
Signaling rate is the number of transitions per second required for the proper data rate. Data rate is in bits per second; signal rate is in transitions per second (baud). With some codes, one symbol is all that is needed for one bit, for example, NRZ (Non-Return to Zero); other codes may require two transitions per bit, for example, Manchester, Biphase, and so forth.
2. Bandwidth (Fiber and Link)
Bandwidth is expressed in units of Hertz (cycles/ sec) and is abbreviated as Hz. The band width of a fiber is related to a phenomenon known as dispersion. The dispersion is described in terms of nanoseconds per kilometer. If a zero rise-time signal is introduced at the transmitter end of the fiber, it emerges at the receiver end with a rise time determined by the length of the fiber in kilometers multiplied by the number of nanoseconds per kilometer of dispersion.
Link bandwidth includes the effects of bandwidth limitation in transmitters and receivers.
3. Launched Power (Transmitter)
The power launched into a fiber is directly dependent on the intensity (brightness) projected onto the face of the ferrule at the cross-sectional area of the fiber core. Other factors also affect the coupled optical power into the fiber. They include the numerical aperture of the fiber, material interface differences, and so forth. It is important to note that the launched optical power specification is for usable optical power already inside a specified type of fiber.
4. Dynamic Range (Receiver)
Dynamic range is the difference between the overdrive (dBm) and the under-drive (sensitivity, dBm) conditions for a fiber-optic receiver. The range depends on certain operating conditions, for example, acceptable error rate and signal-rate distortion.
5. Attenuation (Fiber)
Attenuation describes how rapidly power diminishes from a combination of scattering, absorption, and bending losses as it travels through the fiber-optic cable. This property limits the distance that the signal can travel before the power is less than the minimum required for proper receiver operation. Attenuation is expressed in dB per kilometer, that is, it decreases exponentially as it travels through the fiber.
6. Optical Connections (Transmitter and Receiver)
In addition to specialized connectors, such as the
Avago snap-in connector, other options are recognized as standard. These are described in the data sheet with enough detail to ensure compatibility with the connector system to be used. Some of the standard designators are FC, FDDI, MT-RJ, SC, SMA, and ST.
For some of these, only one fiber size is used while others have options for fibers of various types and sizes.
43
Interpreting a Fiber-Optic Data Sheet
The arrangement of Avago’s data sheets for both plastic and glass fiber-optic links is very similar. Included are the key parameters and specifications, test conditions and applications. Shown below are generic specifications for a variety of products.
General Specifications
1. Recommended Operating Conditions
Lists the operating supply voltage needed for the receiver and the currents at which the transmitter should be operated.
2. Peak Forward Current
The maximum amount of current permitted in the LED of the transmitter. The duration of time that the current is at this maximum is limited by other factors of the device, such as the amount of heat that the electrical power generates.
3. Fanout (TTL)
Indicates how many standard transistor-transistor logic device inputs can be connected to the output of the receiver.
4. Minimum and Maximum
The guaranteed limits of performance. Typical refers to the device performance when it is at room temperature (25ºC) and nominal operating conditions.
5. Duty Factor
A measure of the average length of time a signal is high versus low. A duty factor of 50% means that the signal is equally up and down in the same amount of time, over a long time. Duty factor can be adjusted to permit more peak LED transmitter current for more distance capability of a link at the compromise of on versus off time for the LED.
6. Numerical Aperture
Describes a characteristic of the optical port in the fiber, transmitter, and receiver. This characteristic is useful when using the transceiver with cable that has a different numerical aperture than the Avago component.
7. Table of Absolute Maximum Ratings
Lists operating limits for the transmitter and receiver. Supply voltage is the major consideration and should not exceed the specified value or a receiver may fail to function or be damaged. Many links are specified to operate at 0ºC and above. This prevents moisture from freezing in the optical port,
8. Short- and Long-Term Bend Radii (Table of Absolute
Maximum Ratings)
States the specific limits. Having sharper bend for a long period of time will cause plastic cable to cold flow and the cross section to change from circular to elliptical. The latter shape causes higher light losses in the fiber. Long-term bend may also cause static fatigue fracture.
9. Table of Connector Mechanical/Optical Characteristics
Aids the designer in preventing damage or abuse to the cable, connectors or optical ports of the transmitters and receivers.
10. Optical Port Diameter
Measurement of the light at the plane of the glass fiber face that the LED projects through the lens system of the transmitter port. The value in the table helps determine how much of the light couples into the fiber core. This value can be used to calculate a partial amount of coupled optical power into a fiber with a different core diameter.
11. Receiver Input Optical Power Minimum (Table of Electrical/Optical Characteristics)
Eye Center indicates the sensitivity of the receiv-
Optical Power timing characteristics are presented per definitions specific to the product.
12. Bandwidth-Length Product
An alternate means to describe dispersion.
BW*L(MHz*km) =
350
Dispersion ns km power loss.
44
Glossary
10base-2
An approved IEEE 802.3 standard for 200-m links using coaxial cable. The applications are a 10 Mb/s basebandmodulated LANs for HUB or concentrator-to-desktop links.
Ambient Temperature (T
A
)
The temperature of a medium such as air, water, or earth into which the heat of the equipment is dissipated.
T
A
is usually room temperature and is normally assumed to be 25°C if not otherwise specified. The temperature of the surroundings.
10base-5
Same as 10Base-2 but used with 500-meter links.
Amplitude Modulation
10base-FL
An approved IEEE 802.3 standard for 2-km links with 0.85-m LED sources and 62.5/125 um multi-mode
LANs using repeater links to hubs or concentrators. This standard uses the CSMA/CD protocol. With Manchester coding, the signaling rate is 20 MBd.
Conveying information by altering or modulating the strength (amplitude) of a radio signal.
AM may be either analog (continuous) or digital (discrete). In digital AM, the presence of a signal sends a “1”, the absence sends a “0”.
AM radio is an example of a system that uses amplitude modulation.
10base-T American Standard Code for Information Interchange (ASCII)
An approved IEEE 802.3 standard for 100-m links using unshielded twisted-pair wire (UTP). The applications are a 10 Mb/s baseband-modulated LANs for HUB or concentrator-to-desktop links.
A standardized set of alphanumeric fonts. The associated numeric code used to represent fonts inside a computer.
Analog
Access LAN
Signals which make use of electrical analogies, for
The front-end LAN used to interconnect distributed stations and provide access to a backbone LAN, usually by means of bridges or routers, which are located in a wiring closet.
continuous signal rather than a pulsed signal (Compare to Digital).
Anode
Acceptance Angle
The maximum angle with respect to the fiber axis at which an entering ray will experience Total Internal Reflection (TIR). Also see TIR and NA.
The part of the diode that when positive with respect to the cathode causes forward current flow.
$ QRGH &DWKRGH
Acceptance Cone
A right circular cone whose included angle is twice the Acceptance Angle.
)RUZDUG&XUUHQW)ORZ
DQG&KDUJHV
Active Area
The sum of the core areas of the individual fibers in a bundle, as distinguished from the total area of the cable or bundle. Also see Packing Fraction.
ANSI
American National Standards Institute. The coordi-
Alignment, Optical
Lateral and angular positioning of the central axis of a fiber terminus to an optical port to minimize coupling losses.
United States. Current ANSI standards for Fiber Optics include FDDI and Fiber Channel.
Ambient Light
The light from the surroundings (the sun, fluorescent and incandescent lamps, and so forth).
Angstrom
Unit of length formerly used to describe optical wavelengths. The preferred unit now is the nanometer, which is equal to ten Angstroms.
45
Aperture
The diameter of the largest beam of collimated light that can enter or leave the optical fiber, the lens or other optical device.
Note: “Aperture” and “Numerical Aperture” are not related.
Bellcore (Bell Communications Research)
Created in 1984 following the breakup of AT&T, Bellcore does R&D for the seven RBOCs (Regional Bell Operating Companies). Bellcore performs basic commu-
Area Loss
The loss of optical power (usually in dB) resulting when optical is coupled from a large-core-diameter fiber into a small-core-diameter fiber.
Arithmetic Logic Unit (ALU)
One of the three essential components of a microprocessor (the other two are the registers and the control block).
The ALU adds and subtracts; the logic unit performs operations such as ANDing the contents of two registers, or masking (blanking or inhibiting) the contents of a register.
Asynchronous
Not synchronized to a single, stable, time reference.
Asynchronous Transmission
Transmission of data through start and stop sequences without using a common clock signal.
Attenuation
The optical power loss per unit of fiber length caused per kilometer (dB/km) or decibels per meter (dB/m).
Avalanche Photodiode (APD)
A photodetector used in high-speed (broad bandwidth) fiber-optic systems. The avalanche feature results from the rush of electrons across a junction under a very high reverse-bias state. The APD has a much higher cutoff frequency and internal photo-current multiplication than a PIN photodiode; so it is more sensitive at high frequencies.
Bandwidth, Optical
The frequency at which modulated optical power is down
3 dB (1/2) relative to lower frequencies, as the signal is passed through a fiber of given length. Bandwidth varies inversely as the first power of the cable length.
Baud
A unit of measure for the rate of digital signaling, exfor telecommunications equipment. Most notable are
Bellcore 253 (SONET) and Bellcore 983 (components reliability).
Bend Loss
Attenuation caused by bending the fiber optic cable around a restrictive radius of curvature.
Bend Radius
The radius taken by the axis of the fiber cable when it is bent. A minimum bend radius is the minimum radius to which a fiber cable can be bent without exceeding maximum stress in its fiber.
S =
E 6 r r
S = Stress
E = Young’s modulus = 10,000 kpsi for glass r = fiber radius for single-fiber cable
Δr= distance from cable axis to the outermost fiber axis
Bias
The DC operating voltage or current or both applied to a component. Alternately, bias may refer to the circuitry used to achieve the DC operating point. Such circuitry may be passive (consisting primarily of resistors) or active (using a device with gain, such as a transistor or MOSFET).
Binary Coded Decimal (BCD)
A number system in which each nibble (group of four bits) represents a coded decimal character. The decimal number 69 becomes 0110 1001 in BCD. Also known as the 8421 Code.
Bi-Phase Code
A transmission code in which each bit interval be-gins with either a low-to-high or a high-to-low level transition. If the data bit to be encoded is a logical “1” then another transition must occur before the end of the bit interval. If the data bit is a “0” there is no transition until the beginning of the next bit interval.
codes requiring only one transition per bit, the data rate in bits per second (bps) is numerically equal to the signaling rate in transitions per second (baud).
Note: The term “baud rate” is incorrect, because “baud” is the unit of measure for transitions per second or signaling rate.
Either of these biphase codes can be inverted without inverting the data. They are also self-clocking because each bit cell has a transition at the beginning, called a clocking edge. The duty factor is 50% because the offset from a bit without a mid-cell transition is cancelled by the next bit without a mid-cell transition. Because the code requires two transitions per bit, the data rate in bits per second is half the signaling rate in baud.
46
Bit
An abbreviation for binary digit. A bit is the smallest unit of information that a computer uses. A bit can have one of two values, generally represented as 0 or 1 or, in electrical terms, “on” or “off”. Computers calculate by using binary
(base 2) arithmetic, which involves rapidly manipulating strings of bits according to certain fixed rules.
Clock
A device that provides a continuous series of alternating 1’s and 0’s to control the timing of bits sent in a data stream and controls the timing of the sampling of bits received in a data stream.
Bit Error Ratio (BER)
In an actual transmission system, various electronic noise sources can change or corrupt the stream of data bits.
The number of these corrupted bits divided by the total number of received bits within an arbitrary time period is the bit error rate (BER). The lower the BER value, the fewer the number of errors in the transmission.
Computer System
A computer and software plus one or more pieces of peripheral equipment.
Continuous Wave (CW)
An electromagnetic signal consisting of waves having successive oscillations that are identical under steady state conditions, as opposed to a pulsed signal, consisting of waves that vary with time.
Breakdown Voltage
The voltage measured at a specified current in the breakdown region of a semiconductor diode at which there is substantial change in characteristics from those at lower voltages.
Byte
Eight consecutive bits treated as an entity. Also see Bit.
Control Bus
A device that conveys a mixture of signals, which regulate system operation. These “traffic” signals are commands that may also originate in peripherals for transfer to the CPU or in the CPU for transfer to the peripherals.
Cable, Optical
A cable containing plastic or glass fiber(s) used as a waveguide to carry light waves. Each fiber has three well-defined regions: the core, which is surrounded by the cladding, which in turn is surrounded by tensile strength members and an outer scuff-resistant jacket or sheath.
The cable may contain a single fiber or many fibers. Also see Fiber Bundles.
Cathode
The part of the diode that, when negative with respect to the anode, causes forward current flow. (see
Anode) Central Processing Unit (CPU)
The brain of any computer system. The CPU consists of storage elements called registers, computation circuits in the ALU, the Control Block, and I/O. As soon as LSI technology was able to build a CPU on an IC chip, the microprocessor became a reality. The one-chip microprocessors have limited storage space, so memory is added in modular fashion. Most current microprocessors consist of a set of chips, one or two of which form the CPU.
At incidence angles less than the critical angle, only partial reflection occurs. Incidence and re-fraction angles are measured from a line perpendicular to the boundary.
Also see TIR, and Snell’s Law.
Curvature
A smooth change in the direction taken by a fiber cable. The minimum curvature radius is limited by the actual fiber strength. Curvature causes optical radiation losses.
Daisy Chain
A bus line that connects devices in series by means of active repeaters.
Data Format
(see Transmission Code)
Cladding
Silica (glass) or plastic that surrounds the core of an optical fiber to minimize surface scattering loss-es and establish the NA (Numerical Aperture) for Total Internal Reflection to occur.
Core
The center of the optical fiber through which the transmitted light is propagated. It has the highest refractive index of the fiber.
Critical Angle
The incident angle within a high-index medium that causes the exit angle of a light ray into the second medium to equal ninety degrees.
47
Data Rate
Bits of information (data) transmitted per second depending on the transmission code used, for ex-ample, RZ
(Return-to-Zero), NRZ (Non-Return-to-Zero). The data rate is affected by the data for-mat. RZ delivers a lower data rate than NRZ. If a system’s signaling rate is limited to a high of 10 Bd, a higher data rate may be achieved by dividing the data stream among additional channels.
Data Rate (Mb/s) = Signaling Rate (Mbaud) x Efficiency of Transmission Code
Also see Baud.
dBc
Decibels referenced to the signal carrier level.
dBm
Decibels referenced to 1 mW. The standard unit of power level used in microwave work.
Detectivity
A parameter of a photodiode. Detectivity characterizes the normalized area of a photodiode surface by normalizing the signal-to-noise ratio with respect to the square root of the area and the incidence (optical power per unit area).
Detector, Photo
A device that reacts to light by producing or modulating an electrical signal. Also see Photodiode, Pin Diode and Avalanche Photodiode.
Dispersion
The increase in rise/fall time of the optical signal at the far end of long fiber-optic cables. It is expressed in nanoseconds per kilometer. Dispersion is linearly related to the inverse of the bandwidth-times-length constant. It results from either modal or material effects. Also see Modal Dispersion and Material Dispersion.
dBm mW
0 1
10 10
20 100
Also see dB.
Digital
Pertaining to data in the form of discrete quantities or digits. Also see Analog.
Diode
An electronic device composed of two different mixtures of semiconductor materials that allows large currents to pass in only one direction.
DC (Direct Current)
A unidirectional current in which the changes in value are either zero or so small that they may be neglected.
Debugging
The process of removing errors from a computer program so that it executes instructions properly.
Directional Sensitivity
The off-axis response of a detector.
Doping
Adding impurities to a substance to change its electrical properties.
Decibel (dB)
A unit of gain equal to ten times the common (base
10) logarithm of the ratio of two power levels or 20 times the common logarithm of the ratio of two voltage or current levels, as shown in the following table.
dB
Dual In-line Package (DIP)
A housing for integrated circuits with two rows of pins.
Duty Cycle
The ratio of on time to off time in repetitive operation, generally expressed as the on percentage of total cycle time.
Duty Factor
9 x8.00
When an LED is rapidly pulsed On and Off, the duty factor is the ratio of On time to total On plus Off time.
DF =
On
On + Off
Decoder
A circuit or device that automatically converts a signal to the appropriate switching action.
48
Electro-Magnetic Capability (EMC)
The ability of electronic equipment to be operated in the intended electromagnetic environment at designed levels of efficiency. Fiber Optics helps solve EMC problems.
Electro-Magnetic Interference (EMI)
An electromagnetic disturbance generated within or external to electronic equipment that impairs the reception of a desired electromagnetic signal.
Forward Voltage (VF)
Voltage across a diode for a given forward current.
Frequenc y (f)
The repetition rate of a periodic signal, expressed in cycles per second. The units of frequency are Hertz (Hz).
Common multipliers include:
Typical sources could be power-line transients, noise from switching-type power supplies or spurious radiation from oscillators or both. EMI is suppressed by power-line filtering or shielding.
Electro-Static Discharge (ESD)
The instantaneous release of stored electricity from one body to another. Fiber optic transmission is immune to ESD.
• kHz (kilohertz): 10 3 Hz
• MHz (megahertz) : 10 6 Hz
• GHz (gigahertz): 10 9 Hz
Most Avago FCD products are designed to operate in the
MHz to GHz frequency range.
Frequency Range
The minimum and maximum frequencies between which a particular component will meet all guaranteed specifications. Alternatively, the minimum and maximum frequencies between which a particular component will have “useful” performance.
Emitter, Optical
A device that generates optical power. Also see ILD, LED, and Light Source.
Full-Width Half-Minimum (FWHM)
(see Spectral Width)
Extinction Ratio Gain
The ratio of the output power of a digital transmitter for a logic 1 state compared to a logic 0 state. This ratio is important in system design because it affects the recovered signal level at the receive end. Laser transmitters are usually designed with a compromise between switching speed and extinction ratio performance.
The amplification available from a component expressed as the ratio of the output power to the input power. Gain is usually measured in decibels (dB).
Graded-Index Fiber
Fall Time
An optical fiber whose index of refraction decreases as a function of the distance from the center of the core, until it reaches the cladding index of refraction at the core radius. Graded-index fibers offer multi-mode On the trailing edge of a pulse, the time it takes for the instantaneous power to decrease from 90% of the peak pulse power level to 10% of the peak pulse power level.
Fiber Optics
and are used in all fiber applications. It is one of the two most popular fibers, the other being Step-Index Fibers.
See also Index Profile.
The technique of conveying light through a particular configuration of glass or plastic fibers.
Hertz (Hz)
Fiber Strength
A unit of frequency equal to one cycle per second.
(See Tensile Strength)
High-Order Mode
Filter
(see Modes of Propagation)
A device that rejects signals of certain frequencies while passing others.
Flux Budget
(See Optical Power Budget)
Hole
A vacant, electron energy state apparent as a charge defect in a crystal. This defect behaves as a positive charge carrier, equal to the charge strength of an electron.
Forward Current
Index Grading Coefficient (g)
Current through a diode in the direction of its maximum conduction.
Describes the variation of refractive index within the fiber core as a function of radial distance, r, from the center of the core, relative to the core radius, a.
49
Index Profile
The index of refraction through the fiber core as a function of the distance from the center of the core. A sharp reduction of the index at the boundary between core and cladding results in a step-index fiber. A gradual lowering of the index in the core areas approaching the cladding results in a graded-index fiber. (Also see
Index Grading Coefficient)
Index of Refraction (n)
The ratio of the velocity of light in a vacuum (such as the fiber core, n, or cladding, n) to the velocity of light in a given medium. (Also see Snell’s Law)
Ionization
The process of giving net charge to a neutral atom by adding or subtracting an electron. Can be accomplished by radiation, or by creation of a strong electric field. Junction
Infrared (IR)
Wavelengths that extend beyond 770 nanometers.
Injection-Laser Diode (ILD)
A p-n junction semiconductor that converts direct
The region between semiconductor layers of opposite polarities.
Lambertian Emitter
An emitting area whose luminous intensity varies directly as cos θ varies (constant sterance).
optical output power. The ILD provides a light source suitable for optical fibers. It can supply much higher optical power output in a smaller NA and smaller spectral width than LEDs, but is costly and temperature dependent.
Lambertian Source or Reflector
A source or reflector whose numerical aperature (N.A.) is unity.
Input/Output (I/O) Data Lines
Package pins which are tied directly to the internal bus network to enable an I/O to interface the microprocessor with other components of the system and the outside world.
Light-Emitting Diode (LED)
A p-n junction that emits light when biased in the forward direction.
Insertion Loss
A loss resulting from the insertion of a component in a system, the ratio of the power delivered to that part of the system following the component to the power delivered to that same part before insertion. Insertion loss is typically measured in dB.
A semiconductor device that provides a spontaneous (non-coherent) light source through the process of electroluminescence. LEDs emit visible or invisible
(infrared) radiation. LEDs are suitable for optical fibers when adapted to match the fiber’s core area. This interface may be in the form of a pigtail fiber lead attached to the light-emitting slide.
Integrated Circuit
A circuit with many elements fabricated on one piece of semiconductor substrate material. Abbreviated IC.
Light Sources
Injection Laser Diodes (ILDs) and Light Emitting
Diodes (LEDs). ILDs are capable of coupling a few mW of light power into an optical cable; LEDs can couple only a few hundred μW. (Also see ILD and LED)
Intensity (Iv)
Number of flux lines per unit solid angle.
Linear
A device whose output is directly proportional to its input.
Interface
1. A surface forming a common boundary between adjacent regions.
2. A common boundary between adjacent components, or systems enabling the devices to yield and/or acquire information from one another.
International Electrotechnical Committee Regulation No. 825
(IEC 825)
The standards body in Europe that sets general standards and safety regulations for various electrical products. IEC 825 sets the eye safety optical output power limits for laser- and LED-based devices. Similar in concept to the CHRH in the USA, the IEC has specific regulations that may cause different eye safety classification.
50
Linear Dynamic Range Manchester Code
The linear dynamic range is determined by three parameters of the amplifier:
- noise floor
A modulation code that has a transition in the center of each bit cell. The transition is low-to-high for a “0”, highto-low for a “1”. Successive “ones” or “zeros” require additional transitions at the ends of the bit cells.
- gain of the amplifier
- linear output power
The range between the noise floor and the 1 dB gain compression point is the linear dynamic range.
The lowest input signal level that produces a detectable output is determined by the thermal noise generated within the amplifier itself. Any signal below this
“noise floor” causes an amplifier output with a signal-tonoise ratio of less than one, which requires special techniques to recover useful information. The noise floor may be calculated from:
This code is self-clocking because each bit has a midcell transition called a clocking edge. The duty factor is 50% because the second half of each bit cell has a polarity opposite that of the first half. The code is not invertible because the mid-cell transition is polarized. It must be low-to-high for a “0” so that inverting the signal also inverts the data.
Because the code requires two transitions per bit, the data rate in bits per second is half the signaling rate in baud.
Material Dispersion
Pni (dBm) = -114 dBm +10 log B + NF
Pni = effective input noise power in dBm
The separation of the light wave into its component frequencies over a specified time period as it travels through the fiber. This phenomenon limits bandwidth in fiber-optic cores. It is caused by two conditions:
B = noise bandwidth of amplifier or system in MHz
NF = amplifier or system noise figure in dB
Note:
Noise bandwidth can be approximated by the 3dB bandwidth of the amplifier gain response. The published bandwidth of most
Avago amplifiers is not the 3dB bandwidth, but is the flat gain response region. It is suggested that bandwidth be limited by external filtering or system selectivity.
1. The velocity of optical transmission in the core varies inversely as the index of refraction.
2. The index of refraction varies according to the wavelength spectrum.
This means that although all portions of the optical signal may be launched simultaneously, they will not
When performing sensitivity calculations, it is all arrive simultaneously. Due to differences in travel usually assumed that the actual minimum detectable time, some portions of the spectrum will arrive at a differsignal (MDS) is some level above the noise floor (typical ent time (that is, be dispersed).
value is 3 dB).
Mean Time Between Failures (MTBF)
Linearity
Any deviation from a best-fit straight-line approxima-
A calculated figure representing the estimated average lifetime of a repairable device before it fails.
level (IM level), spurious content, compression, and intercept point.
Mean Time To Failure (MTTF, MTF)
A calculated figure representing the time at which 50% of a population of devices will have failed. MTTF is used to predict the reliability of non-repairable devices such as individual semiconductors.
Lumen
A basic unit of measure used for the total amount of light flux in any particular situation; for example, from a source, in a beam, through an aperture, and so forth.
Luminous
Relating to how the human eye sees the light.
Luminous Efficiency
The ratio of light output to power input.
Microprocessor
A Central Processing Unit fabricated on one or two chips.
While no standard design is visible in existing units, a number of well-defined, separate areas are present in all of them: Arithmetic and Logic Unit, Control Block, and
Register Array. When joined to a memory storage system, the resulting combination is referred to in today’s usage as a Microprocessor.
Luminous Intensity
A measure of the visibility of a light source generally expressed in millicandelas. It is defined as lumens per unit solid angle (steradian) .
51
Miller Code
A modulation code in which each logic “1” has a transition at the center of the bit cell, and a logic “0” has a transition at the beginning of the bit cell, unless the “0” follows a “1” (compare with Manchester Code) Note that the
Miller code has a transition (either rising or falling) corresponding to each rising edge in the Manchester code.
Common Fiber-Related Modulations
Type Description
AM Amplitude Modulation - information is passed by varying the signal amplitude
The Miller code has a duty factor that ranges from 33 to 67%, but is typically about 50%. It can be self-clocking with a phase-locked oscillator. Its main advantage is that it requires only one transition per bit, making the data rate in bits per second equal to the signaling rate in baud.
Also, inverting the signal does not invert the data.
Modal Dispersion
The expansion of the rise and fall times of the signal as it travels along the fiber, expressed in ns/km. The time of arrival of simultaneously transmitted flux (optical radiation) depends on the path length traveled. Since reflections of the flux rays occur at different angles, path lengths will vary, causing dispersion. Also referred to as spreading. Modal dispersion is greatly reduced by use of fully graded fiber and reduced to zero in singlemode fiber. Also see Material Dispersion.
FM Frequency Modulation - information is passed by varying the signal frequency
PM Phase Modulation - information is passed by varying the phase of the signal
Modulator, Optical
Light sources such as LEDs and ILDs can be modulated directly by varying the drive current (100 to 300 mA) at a data rate from DC to 150 Mb/s NRZ (for LEDs) and beyond
(for ILDs).
Monomode Fibers
(see Single-Mode Fibers)
Multi-mode Fibers
Single fibers that can accommodate both high-order large NA.
Modem Multiplexer
Abbreviation for modulator-demodulator, a device that couples a computer or terminal to a telephone line. In general, any device that modulates an out-going
A device that accepts a number of parallel inputs and serializes them for transmission to a remote demultiplexer where they are converted back to parallel signals.
Multi Quantum Well (MQW)
ing signal to proper format. Used for long-distance data transmission.
Modes of Propagation
The MQW structure is a change to basic laser physics that improves a laser’s operation. The practical advantage to the MQW is that it typically has more stable operating characteristics over its temperature range.
Optical rays may propagate at various angles with respect to the fiber axis. The smaller angles are called loworder modes and the larger angles are called highorder modes. Both classes of modes are totally reflected within the core.
Multiplexing
The process of combining two or more channels of information for transmission on a single channel.
Mode Mixing
Noise Equivalent
Conversion of low-order modes to high-order modes due to bends in the fiber.
An input signal that produces an output signal level that is equal in value to the noise level present when no signal is applied.
Modulation
Noise Equivalent Power (NEP)
Adding information to a carrier frequency by varying some combination of signal amplitude, signal phase, and or signal frequency.
Modulation may be either analog (continuous) or digital (transmission of a finite number of discrete states).
The input signal power that produces an output signal level equal in value to the noise level present when no signal is applied. This noise equivalent in watts is called the Noise Equivalent Power.
Devices for photon detection can be compared on the basis of NEP.
NEP =
Noise Current (amps per square root Hz)
Opti c a l Power Responsivity (amps per Watt)
52
Non-Return-to-Zero (NRZ)
A transmission code or data format in which the signal level does not periodically return to zero between a succession of high or “1” bits. A clock signal at the receiving end is required to define the point at which each bit of data is valid. A separate channel is required to transmit the clock signal.
Numerical Aperture (NA)
A number that expresses the angular light-gathering characteristic of an optical fiber. It is expressed by the sine of the acceptance angle, the maximum angle with respect to the fiber axis at which an entering ray will experience total internal reflection (TIR).
Optical Power Responsivity
At any particular wavelength and for the flux in a small spot falling entirely within the active area of the photodiode, responsivity is the ratio of the incremental photodiode current to the incremental optical flux producing it.
Optics
The scientific study of light and vision, chiefly the generation, propagation, and detection of electromagnetic radiation having wavelengths greater than X -rays and shorter than microwaves.
Optical Power Responsivity
The ratio of incremental photodiode current to the incremental optical power producing it. Usually expressed in amperes per watt (A/W).
Optoelectronics
Technology dealing with the coupling of functional electronic blocks by light beams. Circuitry in which solidstate emitters and detectors of light are involved.
Phonon
A quantum of heat energy.
Photometry
A branch of science which deals with the measurement of light intensity.
Photodetector
(see PIN Photodiode, Photodiode and Avalanche Photodiode)
Photoconductor
A resistor whose conductance (reciprocal of resistance) increases with exposure to optical radiation.
Photodiode
A solid-state device similar to an ordinary diode with the exception that the light incident on the pn- junction causes the device to produce a current in the direction from cathode, n, to anode, p, rather than in the other direction.
Photon
An elementary particle of light (quantum) with energy hf, where h is Plank’s constant, and f the light frequency.
h = 0.004133 electron-volts per terahertz f(THz) = 300,000/wavelength (nm)
PIN Photodiode
In this semiconductor type, the p-doped and n-doped regions are separated by a lightly doped “intrinsic” region in which the absorbed photons generate an electric current. Hence the acronym PIN. PINs have the advantage of broad spectral response, wide dynamic range, high speed and low noise, but no internal gain.
Point Source
A source of light which emits light in all directions as if from one point. Sometimes used to refer to very tiny light sources.
Packing Fraction
The ratio of the active areas of a fiber-optic bundle to the total bundle area. Only the core areas are considered active; other areas of a cable bundle, including the cladding, do not contribute to coupling efficiency.
Parallel Operation
Processing all the digits of a word or byte simultaneously by transmitting each digit on a separate channel or bus line.
Peak Wavelength
The wavelength at which the output spectrum of an
LED is at a maximum.
Peripheral Equipment
Input-output and data storage devices: printers, key-
Polarized Light
Light in which the electric field points in a known direction.
Port
Device terminals which provide electrical access to a system or circuit. The point at which the I/O is in contact with the outside world.
Power Budget
The ratio (usually given in dB) by which the power provided by a transmitter exceeds the power required at a receiver. Power Budget describes the transmission loss ratio that is tolerable to the transmitter-receiver system.
and tape and disk drivers.
53
Power Budget, Optical
Optical power (light rays) that radiates in a flux of no electrical charge and no magnetic field.
Printed Circuit Board (PCB or PC Board)
A circuit in which the interconnecting wires have been replaced by conductive strips printed or etched onto an insulating board.
Quantum Efficiency
A measure of photodetector efficiency. It is the ratio of primary photoelectrons to photons incident on the detector, expressed as the ratio: “electrons per photon” at a specific wavelength and temperature.
Refraction
The bending of an optical ray incident at a boundary where there is a change of refractive index of the optical material. A fraction of the ray will pass through if the angle of incidence is less than the critical angle.
(called refraction angle or exit angle) that is related to the incidence angle by Snell’s Law.
Refractive Index
(see Index of Refraction)
Propagation Delay
The time difference between a logic transition at the output of a circuit (or device) and the input transition that caused it.
Pulse Dispersion
(see Modal Dispersion, Material Dispersion and Dispersion)
Refresh
To restore information; in displays, to strobe over and over again with the same data.
Register
Computer memory on a smaller scale. The words stored therein may involve arithmetical, logical, or transferral operations. Storage in registers may be temporary, but even more important is their accessibility by the
CPU. The number of registers in a microprocessor is considered one of the most important features of its architecture
Radiant Energy
Relating to the actual energy emitted.
Radiometry
A branch of science dealing with the intensity of radiant energy.
Repeater, Optical
A device that amplifies and reshapes the parameters of an optical signal to agree with the original information and transmits the new signals through a fiber optic line to another repeater or to a receiving terminal.
Random Access Memory (RAM)
Immediate access to any storage location point in the memory by means of vertical and horizontal coordinates. Information may be “written” in (stored) or “read” out (retrieved) in the same rapid way.
Receiver (Fiber Optic)
A device that reacts to light signals, and converts them to electrical signals. Also see Detector.
Resistance (R)
The real (frequency invariant) portion of the Impedance (Z). The basic unit of resistance is the ohm (Ω).
Responsivity
The measure of a photodetector’s performance. The unit of measure is signal current divided by optical power, A/W. Also see Optical Power Responsivity.
Reflection
When an optical ray encounters a change of refractive index, there is a reflection. If the ray is incident on the low-index side, the reflection is always partial. If it is incident on the high-index side, the reflection is partial only for incidence angles less than the critical angle. At incidence angles greater than critical, the reflection is total. The partial reflection is given by:
Return-to-Zero (RZ)
A pulse-type transmission code in which the signal level remains at zero for logic “zeroes,” and momentarily deviates from zero for each logic “one,” returning to zero between successive “ones,” hence the name.
Reverse Breakdown
Phenomenon where large reverse currents flow in a diode due to mechanisms other than normal diode char-
(n n )
2
R =
(n + n )
2
For n < n
2
, the c riti c a l ang l e = ar c sin
(n )
(n ) is exceeded.
Reverse Current
Current flowing through a diode in the direction opposite to the direction of maximum conduction.
54
Reverse Voltage (VR)
Voltage across the diode for a given reverse current.
Rolloff
A gradual decrease in signal strength.
RS-232
Standard wire interface defined for a short-distance connection to a modem data communications equipment (DCE). It is specified at 19.2 kBd for distance up to
15 metres (50 feet).
SDH
Synchronous Digital Hierarchy, the European equivalent of ANSI SONET. (Also see SONET)
Secondary Optics
Any reflector control or lens or both employed to redirect light emitted from an LED package.
Semiconductor
A solid crystalline substance with electrical conductivity greater than an insulator but less than a good conductor.
Single In-Line Package (SIP)
A housing for integrated circuits with one row of pins.
Single-Mode Fibers
Fibers with core areas and numerical apertures so small that only one mode can be propagated through them. Also see Modes of Propagation.
Snell’s Law
At a boundary where there is a change of index of refraction light bends toward the normal when it enters an optically denser medium, and it bends away from the normal as it enters an optically less dense medium.
n
A sinθ
A
= n
B sinθ
B
SONET (Synchronous Optical Network)
A set of standards that allow telecommunications equipment from various manufacturers to inter-operate. SONET specifies standard data rates of OC-1 (51 Mb/s), OC-3 (155
Mb/s), OC-12 (622 Mb/s), and OC-48 (2.488 Gb/s). The data format, signaling and monitoring functions are also specified. In Europe SONET is known as SDH (Synchronous Digital Hierarchy), with STM-1 (OC-3) at 155 Mb/s,
STM-4 (OC-12) at 622 Mb/s, and STM-16 (OC-48) at 2.488
Gb/s.
Spectral Width
1. The incremental energy emitted by a light source as a function of wavelength. Because the Index of Refraction varies over the wavelength spectrum for a fiber, the travel time of different parts of a spectrum will vary, causing Dispersion.
2. The wavelength range over which the performance of emitters, detectors and other optical materials is regarded as being high enough to be useful. between the half-peak levels, and is called FWHM
(Full-Width Half-Maximum).
Spectrum
A continuous range of electromagnetic radiation
(power from a source is not the same at all wavelengths, and the response of a detector varies with wavelength).
Spreading
(See Modal Dispersion)
Step-Index Fibers
Fibers whose index of refraction is reduced sharply at the boundary between the core and the cladding. They are useful for multi-mode operation over short distances up to one kilometer.
Steradian
A unit of solid angle.
Sterance
Intensity per area, usually in millicandellas per square meter.
Strobe/Multiplex
To rapidly pulse an LED On and Off or to rapidly pulse a set of LEDs On and Off in sequence (only one on at any one time), so as to share circuitry and wires.
Substrate
A material that supports other (usually thinner) material.
Tensile Strength
The total mechanical strength of a fiber, and the force in newtons or pounds at which breakage occurs. It is determined by the condition of its surface. Coating the glass fiber with a thin plastic film increases mechanical strength compared to the naked fiber.
Thermal Resistance
Resistance to heat flow.
Condu c ted Heat =
T emperature Differen c e
T herma l Resistan c e
Three-Five (III-V) Compounds
Compounds manufactured from elements in groups have semiconductor properties.
55
Total Internal Reflection (TIR)
Reflections from rays incident on the high-index side of a boundary between materials of different refractive index, in which the incidence angle exceeds the critical angle.
Optical fibers have a core surrounded by cladding with an index lower than that of the core.
Optical rays that approach the wall of the core at incidence angles greater than the critical angle are totally reflected back into the core until they reach the far end of the fiber. Also see Reflection.
Transistor-Transistor Logic (TTL)
A logic circuit design with the diode inputs re-placed by a multiple-emitter transistor. TTL gates using npn transistors are positive-level NAND gates or negative-level NOR gates.
Transmission, Optical
A method of transmitting information by means of modulated light waves through an optical system. The system may be composed of any combination of lens optics, mirror optics, or fiber optics.
Transmission Code
A code used to efficiently transmit data along a link. The speed of the stream of data bits in bits per second depends on the transmission code. The most common codes are known as NRZ, RZ, Manchester, BiPhase, and Miller. Also see Non-Return to Zero (NRZ), Return to Zero (RZ), Manchester and Bi-Phase.
Transmission Loss
(See Attenuation)
Transmitter, Optical
A device that takes electrical signals and converts them to light signals. Also see Light Source and Emitters.
Valence Band
The range of energy states containing the valence electrons that bind crystals together. In a semiconductive material, it is directly below the conduction band.
Viewing Angle
The off-axis angle where the display may be satisfactorily viewed (limited by lenses, obstructions, or intensity decrease).
Wafer
A thin slice cut from a cylinder of III-V compounds. These slices are cut into smaller sections to form chips.
Waveguide, Optical
A transmission line consisting of a plastic, liquid, or glass fiber or bundle of fibers through which light is propagated both axially and by internal reflections.
Wavelength
Length (usually in nanometers) of one cycle of a light wave.
Word
A group of numeric and/or alphabetic characters and symbols, treated as a unit and given a single location in computer memory. Also see Byte.
For product information and a complete list of distributors, please go to our web site: www.avagotech.com
Avago, Avago Technologies, and the A logo are trademarks of Avago Technologies in the United States and other countries.
Data subject to change. Copyright © 2005-2011 Avago Technologies. All rights reserved.
AV02-0583EN - January 5, 2011
advertisement
Related manuals
advertisement